T.N. Boardaa, * L. Yangbb, M. Salehbb
aManchester University, Oxford Road, Manchester, M13 9PL, UK
bDivision of Clinical Sciences (North), University of Sheffield, Coleridge House, Northern General Hospital, Sheffield S5 7AU, UK Accepted 2 December 2005
Abstract
Tensioned fine-wire external fixator systems have been used successfully for the treatment of fractures, mal-unions and for limb lengthening for many years. When used in metaphyseal bone, this type of fixator has a lower loosening rate than half-pin fixators. The differing mechanical properties of these fixator systems have been investigated extensively; however, most studies have centered on the mechanical properties of the fixator as a whole. Our knowledge of the interactions occurring at the interface between implant and bone remains sparse. In order to investigate this interaction, we devised a simple experimental model to characterise the distribution of pressure in cancellous bone surrounding a tensioned wire under loading conditions. Comparison was then made to a similar model of a half-pin fixator. Pressure was measured using pressure sensitive film at various distances away from the implant–bone interface. Static, single cycle loading of the model was performed with a Universal Testing Machine. Pressure distribution in the fine-wire model was found to occur in three regions: polar, beam loading and uniform. Polar patterns were seen closest to the wire with pressure concentrated at the entry and exit points of the wire. Beam loading was seen at a distance of 1.5mm from the wire and pressure reached a uniform distribution at 4.0 mm. Most of the pressure measured was less than 2 MPa, which is less than the yield strength of cancellous bone (2–7 MPa). Higher loads produced higher stresses but the distribution pattern was similar. In contrast, the half-pin model showed far higher pressures (20 MPa), which were present deeper in the bone specimen. These results further our understanding of the biomechanics of fine-wire fixators and may explain the lower loosening rate of this type of fixator when compared to half-pin fixators used in metaphyseal bone.
© 2006 Elsevier Ltd. All rights reserved.
Keywords: Fine-wire; Interface; Pressure distribution; Ilizarov
1. Introduction
Tensioned fine-wire external fixator systems have been used regularly for the treatment of fractures, malunions and limb lengthening since the pioneering work of Ilizarov in the 1950s(Ilizarov and Ledyaev, 1992). The fine wires are flexible 1.5–2.0mm diameter Kirschner wires. They are passed through the bone and either end is fixed to a ring. The wire is tensioned to 500–1300 N. At least two wires are used per ring. This tensioning converts the flexible wire into a relatively rigid structure and is largely responsible for the rigidity of the whole system. The effect is analogous to the bicycle wheel, where the tensioned spokes pull against one another to create a light, strong and rigid composite structure. Wires are also available with olives or stoppers about one third along their length so that bone fragments can be compressed. The uses for these systems is still expanding (Farrar et al., 2001; Saleh et al., 1999) with recent reports including arthrodesis of the knee and foot (Oostenbroek and van Roermund, 2001; Weber et al., 2002), the treatment on Blount’s disease and Madelung deformity (Accadbled et al., 2003; Schroder et al., 2003). The alternative to tensioned fine wires is the use of so-called half pins. These are essentially long screws that are inserted percutaneously into the bone through one cortex. The external fixator is then attached to the shaft of the pin.
shaft of the pin. It has been noted that half pins placed in the metaphyseal area tend to loosen prematurely when compared to their secure fixation in diaphyseal bone (Aro et al., 1993; Lawes and Goodship, 1998; Reed et al., 1998). Conversely, tensioned fine wires have considerable holding power in metaphyseal bone and do not exhibit this early loosening. Similarly, fine wire fixators remain successful when used in patients with relative osteoporosis (Ali and Saleh, 2000; Ali et al., 2003). It has been suggested that metaphyseal half pin loosening is due to the stress at the pin–bone interface (Pettine et al., 1993). One may suppose that if stresses produced at the pin–bone interface are high enough to produce osteolysis and loosening, then the stresses at the wire–bone interface of a circular fixator should be higher due to the lower surface area of the thin wire and loosening should also occur. This is in opposition to observed outcomes with systems such as the Ilizarov and Sheffield ring fixators. The mechanical properties of whole fixator constructs have been well characterised (Yang et al., 2003; Fleming et al., 1989; Podolsky and Chao, 1993; Orbay et al., 1992); however, the interaction between the wire and bone and the pressure distribution at this interface remain un-investigated and may hold the key to understanding the clinically observed differences between these two systems. To investigate this interaction, we devised an experimental model to analyse the distribution of pressure in cancellous bone surrounding a tensioned wire under loading conditions. This was then compared to a similar model of a half-pin fixator.
2. Materials and methods
Cellular rigid polyurethane foam (Type 1522-11, Sawbones Europe) was used to simulate cancellous bone. This foam has a density of 200 kgm-3 and has previously been validated in mechanical tests as having compressive yield strength of 2.71±0.21MPa and compressive modulus of 79.3± 7.55MPa (Thompson et al., 2003). In comparison, cancellous bone has compressive yield strength of 2–7MPa (Li and Aspden, 1997) and a compressive modulus of 54 (SD ¼ 36.7) (Carter and Hayes, 1977). Cellular foams such as this have frequently been used to model cancellous bone in mechanical tests (Gausepohl et al., 2001; Szivek et al., 1993; van Landuyt et al., 1999; Thompson et al., 1997; Stoffel et al., 2004). The artificial cancellous bone was sectioned into multiple blocks of dimensions 65mm x 30mm x 40mm. The length of 65mm was chosen to equate to the width of the human tibia at the level of the proximal metaphysis, a site commonly used for wire insertion.
As a guide for the wire, a 1.5mm hole was machined through each bone block parallel to the upper surface. The depth of this hole from the upper surface was increased incrementally for each specimen to enable determination of the pressure at different distances from the wire. Specimens were produced with the wire surface at d
epths of 0.5, 1, 1.5, 2, 2.5, 3, 4 and 5 mm. A single 150 mm-diameter ring of Sheffield Ring Fixator (SRF, Orthofix SRL, Verona, Italy) was mounted on the base plate of a universal testing machine (UTM, Autograph ASG10kN, Shimadzu Corporation, Japan). A 2mm wire with threaded ends was then passed through the bone specimen and attached to the ring on one side. A previously calibrated strain gauge load cell was passed on to the opposite end of the wire, outside the ring and washers and locking nuts were threaded on to the wire and used create a tension of 1200 N. The strain gauge was used to ensure that the tension on the wire was exactly 1200N before the start of each repeat of the experiment. During the loading cycle, the tension was not adjusted and the output from the strain gauge not recorded. Two-layer pressure sensitive film (Pressurex, Sensor Products Inc., East Hanover, USA) was placed atop the bone block and sandwiched with a further bone block of identical dimensions. A universal joint with a flat plate was attached to the cross head of the UTM and lowered onto the top block. The experimental model is illustrated in Fig. 1. For each set of experimental conditions, the loading cycle was repeated with three different sensitivities of pressure film: Ultra Low (0.19–0.59MPa), Super Low (0.48–2.4 MPa) and Low (2.4–96MPa).
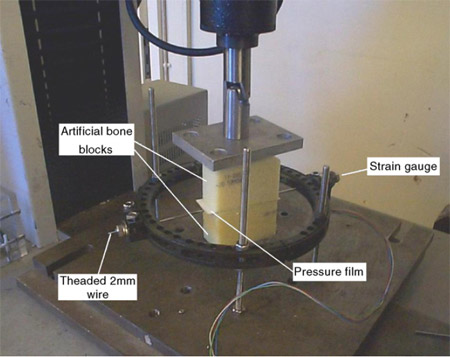
Fig. 1. The experimental model showing the threaded wire and strain gauge load cell. The pressure film can be seen between the two bone blocks.
Two sets of experimental conditions were used. Firstly, the UTM was set to load the bone to 175N for 5 s with a loading velocity of 10 mm/min. The load of 175N was chosen to represent a worst case scenario of a 70 kg patient in a single leg stance on a fracture supported by four tensioned wires where no force is transmitted by the fracture (700N=4 ¼ 175 N). Secondly, a force of 225N was used to represent a larger individual of 90 kg in single leg stance (900N=4 ¼ 225 N). The experiment was repeated three times for each force and each bone block specimen giving 48 sets of results, thereby building up a complete description of the pressure distribution throughout the specimen. The ambient temperature and humidity of the laboratory was recorded for converting the film impression intensity to pressure.
For comparison, a half-pin model was also created and tested. The bone block used in this experiment was identical to that used in the fine-wire model. A pilot hole was drilled in the bone block using a bench lathe. A 6mm diameter stainless steel screw with a thread length of 30mm (110/30, Orthofix SRL, Verona, Italy) was then inserted into the block so that the full thread was embedded in the block. The distance from the surface of the bone block to the top surface of the pin was 3 mm. The pin and block were then attached to the SRF ring with the bone block in the center of the ring using a single screw clamp. A force of 175N was applied at the same rate as in the fine-wire experiment.
The films (Fig. 2) were scanned using a Hewlett- Packard Scanjet ADF scanner onto a PC running Hewlett Packard ScanPro 2.0 software. The scanned images were converted to 16 bit grayscale bitmap files using Abode Photoshop 7.0.1. An analysis program was developed using MATLAB technical computing software (The Mathworks Inc., Natick, Massachusetts, USA). The program converts the image intensity to pressure, according to the manufacturer’s standard and conversion curves, and produces a pressure map. The pressure maps from all the experiments were collated and analysed.
3. Results
3.1. Fine-wire model (175 N)
The pressure maps from all three sensitivities of film show that at a depth of 0.5mm from the wire, there is a concentration of pressure at the entry and exit points of the wire (Fig. 3). We have termed this pattern a ‘‘polar’’ distribution. The pressures recorded near the wire entry and exit sites were seen to reach a maximum of 6–8MPa. The most sensitive film shows slight pressure along the length of the wire but this pressure is less than 2MPa. At the next level, 1.0 mm, we begin to see pressure distribution along the length of the wire although the pressure is still concentrated towards the entry and exits points of the wire. From 1.5 to 3.0 mm, we see an even distribution of pressure along the entire length of the wire, so-called beam loading (Fig. 4). Most of the pressures measured in this region were less than 2MPa. From 4.0mm onwards, the pressure was distributed away from the wire (Fig. 5).
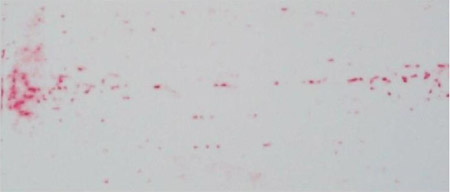
Fig. 2. An example of the developed pressure film.
3.2. Fine-wire model (225 N)
At 0.5 mm, the pattern of pressure distribution is similar to that seen in the 175N series. However, the peak pressures are higher at 15–20MPa. The polar pattern of pressure distribution is seen to progress deeper into the bone, up to 2.0 mm. From 2.5 to 4.0 mm, there is beam loading but the pressures are higher, in the range 0.5–3MPa. Reaching 5.0 mm, a uniform pressure distribution is seen. The three-dimensional graphs from these experiments are not shown.
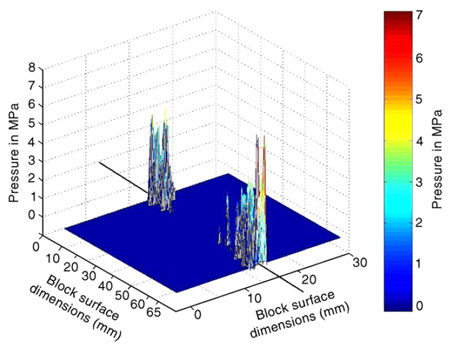
Fig. 3. Three-dimensional pressure map showing path of wire (black line). At a distance of 0.5mm from the wire, there is a polar distribution of pressure towards the entry and exit points of the wire.
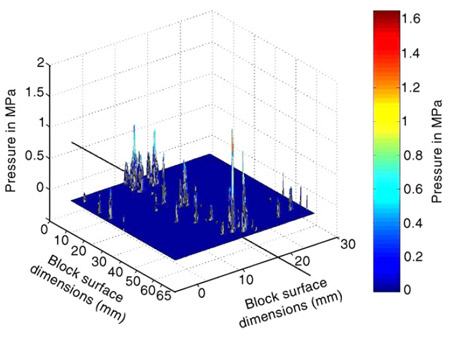
Fig. 4. Pressure map showing beam loading effect at a distance of 2.5mm from the wire (path of wire represented by black line).
3.3. Half-pin model
The pressure distribution seen in the half-pin experiment is entirely different to that described for the finewire experiments. At a depth of 3 mm, where beam loading is seen around a fine-wire, the pressure is grossly concentrated toward the entry point of the pin (Fig. 6). The peak pressures at this depth are observed to be around 20MPa, a factor of 10 greater than the pressures observed at this depth in the fine-wire experiment.
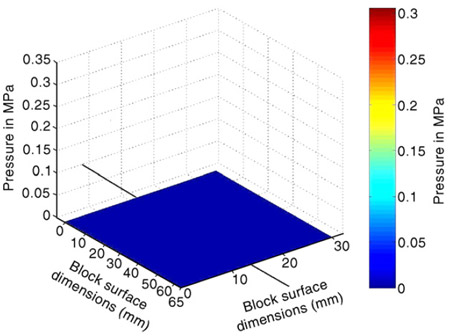
Fig. 5. Pressure map showing uniform pressure at a depth of 5.0mm (path of wire represented by black line)
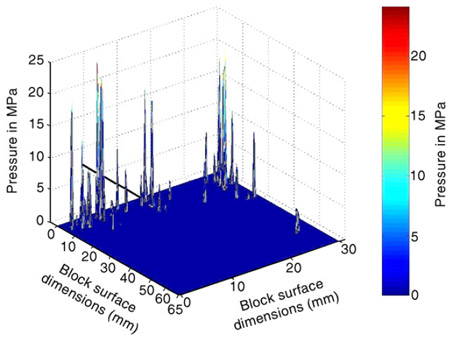
Fig. 6. Half-pin experiment. Pressure map showing concentration of pressure toward the entry side of the pin (black line) at a depth of 3.0 mm. Peak pressures of 20–25MPa are observed.
4. Discussion
This is the first time
that the pressure distribution at the wire–bone interface of a fine-wire fixator has been investigated experimentally. Huiskes et al. (1985) performed a theoretical analysis of the interface stresses in both half-pin and full pin fixators using finite element analysis and parametric analysis. They also report a polar distribution of pressure closest to the wire or pin with the highest pressures at the point where the pin or wire enters and exits the block, which is in agreement with our findings. Interestingly, however, they observe that the pressure did not reach uniform levels until a distance of 10mm from the pin, an observation also made by Manley et al. (1984), in a half pin model. We observed uniform levels of stress at just 5mm from the wire. Huiskes et al. used a diaphyseal bone model with two flat plates of cortical bone and no intervening cancellous bone rather than continuous cancellous bone as in our experiment. We feel that the pressure reaches uniform levels more quickly in our model as there is a larger area of bone to support the wire, hence the pressures are lower and distributed more evenly. Huiskes et al. also note that the interface pressure rises as the pin diameter reduces. They record that the pressure rises in relation to the pin flexibility (a fourth power relation) and the decrease in the bearing surface for the transverse load (a linear relation). This observation does not appear to take into account the pretensioning that is always performed when fine-wire fixators are used. This pre-tensioning has no effect on the bearing surface area but has a significant effect on the pin flexibility. It effectively turns a very flexible wire into a taught, stiff wire. This reduces the wire deflection and therefore reduces the pressure at the interface. Although our experiments were performed at a single wire tension, there is evidence to suggest that on repetitive loading the tension in the wire can fall (Watson et al., 2003; Hillard et al., 1998). This may increase the stresses around the wire and influence the loosening rate if the wire. Further studies are needed in this area.
The polar distribution of pressure close to the wire can be explained by the deflection occurring in the wire on loading. The wire does not bend to a true arc but is seen to undergo a small change of angle as it enters and exits the block. This confirms that the force is being absorbed at these areas of the block. Hence, the pressure concentrations observed here. As the distance away from the wire increases, the pressure distribution gradually becomes one of beam loading. Due to the lattice like nature of cancellous bone, the high pressures near the wire at the entry and exit points are dispersed over a very short distance. The complete length of wire in the block is then able share the load resulting in a beam loading situation. Due to the continuing dispersal of pressure through the bony lattice, at a distance of 4.0mm from the wire the pressure drops to uniform levels. The pattern of pressure distribution seen when the force was increased to 225N was similar to that seen at the lower force. However, the polar pattern was seen to progress deeper into the block. This is predictable from the explanation given above. The deflection of the wire is greater at a higher load, imparting a greater strain on the entry and exit points, leading to a greater pressure. The gradient of the pressure fall is a function of the material properties of the block and is hence equal to the previous model. The higher pressure is therefore transmitted over a greater distance before reaching the levels at which beam loading takes place. The values of pressure recorded in the 175N experiment is below the yield strength of cancellous bone (2–7 MPa) and osteoporotic cancellous bone in most instances (1.5–5MPa) (Li and Aspden, 1997). This explains the low loosening rate seen with fine-wire fixators. However, in the 225N experiment, pressure values greater than this yield strength were observed. These high pressures were only seen at the entry and exit points of the wire and in an in vivo situation even a thin cortex would likely withstand these pressures. The conditions we have chosen to model represent a worst-case scenario that would be uncommon in clinical situations as a significant part of the load is transferred across the fracture site by the time patients start to weight bear.
We have also shown that the pin–bone interface pressures in a half-pin model are much higher (20MPa) than those seen in the fine-wire experiment under similar loading conditions. It should be borne in mind, however, that the interface stress in this situation is more complex than in the wire experiment. A bending moment is present leading to complex shear stresses as well as compressive stress. Clinical experience shows that when half-pin fixators are used in diaphyseal bone with a thick cortex, they are successful as the cortex is able to withstand such high pressures. However, when they are used in metaphyseal regions they fail more frequently by cutting out. We have shown that the pressures at the pin–bone interface of half-pin fixators are much higher than the yield strength of cancellous bone, which may account for the early failure seen in these regions.
One criticism of the experiment maybe that only one wire was used, when in clinical situations two or more wires are used on either side of the fracture. In the clinical situation, one has to construct a three dimensionally stable frame to resist bending in both anteroposterior and medio-lateral directions; therefore, wires are placed at angles as close to perpendicular as anatomical limitations allow. Our experiment was essentially two dimensional: the force was applied perpendicular to the long axis of the wire and the combination of the universal joint of the UTM crosshead and the fact that the bone block could rotate around the wire ensured that there was no element of shear imparted on the wire–bone interface. We believe therefore that, provided the force applied to the wire was appropriate, the addition of extra wires would have no bearing on the results.
We chose to use artificial bone rather than animal bone since we felt that the use of animal bone would introduce unpredictability into the experiment due to the heterogeneity of such bone and variance between samples. The material properties of this foam make it a reasonable model for cancellous bone (Thompson et al., 2003; Li and Aspden, 1997; Carter and Hayes, 1977). Previous studies utilising this type of material have found that when tested to failure, the properties can be quite variable (Thompson et al., 1997). However, in this study, the material did not fail allowing a meaningful comparison between samples. The blocks do not have any form of cortex. This allowed us to model a worstcase scenario of osteoporotic metaphyseal bone. Secondly, had we used a model incorporating a cortex, the number of further variables such as thickness and material of the cortex would have sought to complicate the interpretation of the results. Indeed, the introduction of a cortex to the model would have given us very little information about the interactions at the largest part of the interface, that with cancellous bone.
The present study is one of static, single stroke testing. Clinical situations demand that patients load their fixator multiple times before fracture healing occurs and this may affect the interface stress in a number of ways. Firstly, the wire tension may reduce over time changing the stress distribution and secondly, fatigue failure of cancellous bone may occur at the stresses observed.
This study furthers our knowledge of how ring fixators can safely carry load and effectively distribute pressure in the most inhospitable situations. Although this was a simplified, static, single stroke model the interface pressures around half-pins were found to be higher than
the stresses around tensioned wires. These results improve our understanding of the biomechanics of external fixators.
References
- Accadbled, F., Laville, J.M., Harper, L., 2003. One-step treatment for evolved Blount’s disease: four cases and review of the literature. Journal of Pediatric Orthopedics 23 (6), 747–752.
- Ali, A.M., Saleh, M., 2000. Treatment of bicondylar tibial plateau fractures in the elderly using the Sheffield hybrid fixator. Injury 31, 292–293.
- Ali, A.M., Burton, M., Hashmi, M., Saleh, M., 2003. Treatment of displaced bicondylar tibial plateau fractures (OTA-41C2&3) in patients older than 60 years of age. Journal of Orthopedic Trauma 17, 346–352.
- Aro, H.T., Markel, M.D., Chao, E.Y., 1993. Cortical bone reactions at the interface of external fixation half-pins under different loading conditions. Journal of Trauma 35 (5), 776–785.
- Carter, D.R., Hayes, W.C., 1977. The compressive behaviour of bone as a two-phase porous structure. Journal of Bone Joint Surgery 59A, 954–962.
- Farrar, M., Yang, L., Saleh, M., 2001. The Sheffield hybrid fixator—a clinical and biomechanical review. Injury 32 (Suppl 4), 8–13.
- Fleming, B., Paley, D., Kristiansen, T., Pope, M., 1989. A Biomechanical Analysis of the Ilizarov external fixator. Clinical Orthopedics 241, 95–105.
- Gausepohl, T., Mohring, R., Pennig, D., Koebke, J., 2001. Fine thread versus coarse thread. A comparison of the maximum holding power. Injury 32 (Suppl 4), SD1–SD7.
- Hillard, P.J., Harrison, A.J., Atkins, R.M., 1998. The yielding of tensioned fine wires in the Ilizarov frame. Proceedings of the Institute of Mechanical Engineers [H] 212, 37–47.
- Huiskes, R., Chao, E.Y., Crippen, T.E., 1985. Parametric analyses of pin–bone stresses in external fracture fixation devices. Journal of Orthopedic Research 3 (3), 341–349.
- Ilizarov, G.A., Ledyaev, V.I., 1992. The replacement of long tubular bone defects by lengthening distraction osteotomy of one of the fragments. Clinical Orthopedics and Related Research 280, 7–10.
- Lawes, T.J., Goodship, A.E., 1998. Cortical profile external fixation screws maintain torque in the metaphysis. Journal of Bone Joint Surgery 79 (B suppl), 460.
- Li, B., Aspden, R.M., 1997. Composition and mechanical properties of cancellous bone from the femoral head of patients with osteoporosis and osteoarthritis. Journal of Bone Mineral Research 12 (4), 641–651.
- Manley, M.T., Hurst, L., Hindes, R., Dee, R., Chiang, F.P., 1984. Effects of low-modulus coatings on pin–bone contact stresses in external fixation. Journal of Orthopedic Research 2 (4), 385–392.
- Oostenbroek, H.J., van Roermund, P.M., 2001. Arthrodesis of the knee after an infected arthroplasty using the Ilizarov method. Journal Bone Joint Surgery 83 (B), 50–54.
- Orbay, G.L., Frankel, V.H., Kummer, F.J., 1992. The effect of wire configuration on the stability of the Ilizarov external fixator. Clinical Orthopedics and Related Research 279, 299–302.
- Pettine, K.A., Chao, E.Y., Kelly, P.J., 1993. Analysis of the external fixator pin–bone interface. Clinical Orthopedics and Related Research 293, 18–27.
- Podolsky, A., Chao, E., 1993. Mechanical performance of Ilizarov circular external fixators in comparison with other external fixators. Clinical Orthopedics and Related Research 293, 61–70.>
- Reed, M., Yang, L., Saleh, M., Petrone, N., 1998. Metaphyseal no mans land—does it really exist? Journal Bone Joint Surgery 79 (B Suppl), 462.
- Saleh, M., Yang, L., Sims, M., 1999. Limb reconstruction after high energy trauma. British Medical Bulletin 55, 870–884.
- Schroder, H., Houshian, S., Weeth, R., 2003. Madelung’s deformity treated with Ilizarov-technique, follow-up of 8 cases. Journal of Hand Surgery [Am] 28 (Suppl 1), 34.
- Stoffel, K., Stachowiak, G., Forster, T., Gachter, A., Kuster,M., 2004. Oblique screws at the plate ends increase the fixation strength in synthetic bone test medium. Journal of Orthopedic Trauma 18 (9), 611–616.
- Szivek, J.A., Thomas, M., Benjamin, J.B., 1993. Characterisation of a synthetic foam as a model for human cancellous bone. Journal of Applied Biomaterials 4, 269–273.
- Thompson, J.D., Benjamin, J.B., Szivek, J.A., 1997. Pullout strengths of cannulated and noncannulated cancellous bone screws. Clinical Orthopedics and Related Research 341, 241–249.
- Thompson, M.S., McCarthy, I.D., Lidgren, L., Ryd, L., 2003. Compressive and shear properties of commercially available polyurethane foams. Journal of Biomechanical Engineering 125 (5), 732–734.
- Van Landuyt, P., Peter, B., Beluze, L., Lemaitre, J., 1999. Reinforcement of osteosynthesis screws with brushite cement. Bone 2 (Suppl), 95S–98S.
- Watson, M.A., Matthias, K.J., Maffulli, N., Hukins, D.W., 2003. Yielding of the clamped-wire system in the Ilizarov external fixator. Proceedings Institute Mechanical Engineers [H] 217 (5), 367–374.
- Weber, M., Schwer, H., Zilkens, K.W., Siebert, C.H., 2002. Tibiocalcaneo- naviculo-cuboidale arthrodesis: 6 patients followed for 1–8 years. Acta Orthop Scand 73 (1), 98–103.
- Yang, L., Nayagam, S., Saleh, M., 2003. Stiffness characteristics and inter-fragmentary displacements with different hybrid external fixators. Clinical Biomechanics (Bristol, Avon) 18, 166–172.
*Corresponding author. Tel.: +441706 825969; fax: +441706 824113. E-mail address: [email protected] (T.N. Board).