Femoral Orientation Alters Contact Mechanics in the Hip Joint Under Lateral Trochanteric Loading
D.R. Sparks1, D.P. Beason1, J.E. Alonso2, A.W. Eberhardt1
1Department of Biomedical Engineering, University of Alabama at Birmingham, Birmingham, AL
2Division of Orthopedic Surgery, University of Alabama at Birmingham, Birmingham, AL
Introduction
Side impacts are more likely to cause pelvic fractures than any other type of collision [1]. Among survivors, intra-articular fractures of the acetabulum are especially problematic and secondary osteoarthritis is common. Judet and Letournel [2] concluded that the load path and femoral orientation affected patterns of acetabular fracture. Pressure film techniques have been used to study contact pressures between the femoral head and acetabulum in single legged stance, where the load was applied axially along the femur [3,4]. To date, however, no one has described the contact mechanics associated with seated postures, under side impact conditions.
Presently, we studied the effects of femoral orientation on contact stress localization in the acetabulum, under quasi-static lateral loading through the greater trochanter. We hypothesized that contact areas and pressures would vary with femoral flexion and abduction angles, and would differ from those observed previously for single-legged stance.
Materials and Methods
Nine hip joints were tested from five fresh-frozen cadaver pelves (four males - ages 27, 62, 67, 68 years, and one 73 year old female), obtained through the UAB Willed Body Program. The pelves, which included L4 and L5 vertebrae and the proximal femurs, were cleaned of skin, muscle and remaining viscera. The hip joints were disarticulated to apply pressure film (Pressurex) to the femoral head [3]. The film was cut into rosette patterns with irregularly shaped petals to minimize crinkle artifact. The rosette dimensions were calculated using established equations [5,6]. Low grade (2.4 MPa - 9.6 MPa) pressure film was used to capture the magnitude and location of contact area, mean pressure and maximum pressure. The rosettes were applied to the femoral head using a latex-film-latex system in order to protect the film from moisture. The film was marked at femoral and acetabular landmarks to permit accurate orientation after removal.
To produce the necessary femoral orientations, an apparatus was built allowing variable positioning of the joint in flexion/extension and abduction/adduction. The apparatus fit within an MTS Mini-Bionix (Figure 1). Loads of approximately 930 N were applied over a sixty second ramp through the greater trochanter and held for sixty seconds to allow for complete stain development. Upon unloading, the film was removed from the femoral head. The process was repeated for a combination of three angles of flexion (80°, 90°, 100°) and three angles of abduction/adduction (10° abduction, neutral, 10° adduction).
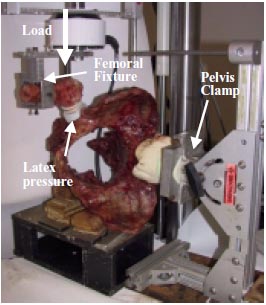
Figure 1 - Pelvis in fixture at 90 degrees flexion, 10 degrees abduction
The stained films were digitized using a flatbed scanner. Each stain was outlined using a tracing function and its area was measured using SigmaScan software. The stains were grouped by location (inferior, anterior, superior or posterior) as shown in Figure 2. Stain density was measured using Bioquant software and mean pressures were determined using our own second-order calibration curve of pressure versus stain density. Contact area (A), mean pressure (P) and contact force (F = P·A) were grouped by quadrant and examined for their association with femoral flexion/extension and abduction/adduction angles using multiple regression (Statview).
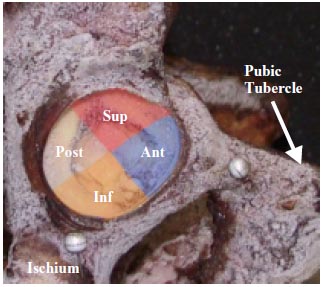
Figure 2 - Contact parameters were grouped according to superior, inferior, anterior or posterior quadrant
Results
The resulting pressure stains showed no signs of crinkle artifact. Comparing contact areas, mean pressures, and contact forces, the most striking trends were observed in the anterior and superior regions of the acetabulum. Figure 3 shows mean contact pressures for the various femoral positions. The multiple regression analyses indicated that changing femoral orientation from 10° abduction to 10° adduction was associated with significant decreases in anterior contact area (p < 0.002), pressure and force (p < 0.0004), and significant increases in inferior contact pressure and force (p < 0.03). Superior contact area, pressure and force significantly decreased with increased flexion (p < 0.02) and increased with increased adduction (p < 0.007). Total contact area and force were not significantly affected by changes in flexion or abduction angle (p > 0.05), which supports consistency between tests.
Discussion
The results indicated that the contact areas and pressures fluctuated with changes in adduction and flexion angles, in support of our hypothesis. The inferior region carried a substantial portion of the applied load, along with the superior and anterior regions of the acetabulum. These findings demonstrate that acetabular contact in lateral loading is notably different than that observed previously for single leg stance, in which the posterior region of the acetabulum was substantially loaded [4,5].
The results of our pressure film study provide direct support for the theoretical predictions of Judet and Letournel [2] that, with increased adduction, “the impact affects increasingly the roof of the acetabulum.” Flexion angle played less of a role in the resulting contact patterns under lateral loading. Our findings are not consistent, however, with the observation that with increased abduction, the main regions of contact shift inferiorly [2]. On the contrary, we observed a significant increase in inferior contact pressure with greater adduction, accompanied by an insignificant increase in inferior contact area. Such variations in acetabular contact may affect the likelihood and type of pelvic fracture under side impact conditions.
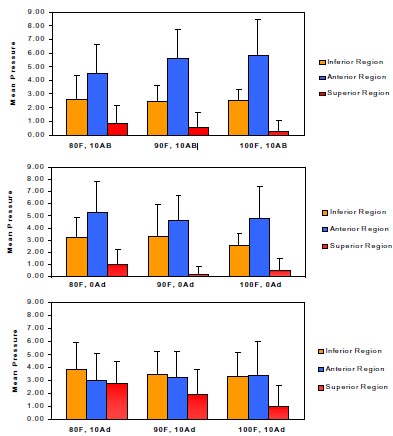
Figure 3 - Contact Pressures for varying angles of flexion and abduction
Acknowledgements
The authors gratefully acknowledge support from the National Highway Traffic Safety Administration and the UAB Center for Injury Sciences.
References
- Gocken, E. C., Burgess, A. R., Siegel, J. H., Mason-Gonzalez, S., Dishinger, P. C., Ho, S. M. (1994). Pelvic fracture mechanism of injury in vehicular trauma patients. Journal of Trauma, 36(6). 789- 795.
- Letrournel, E. and Judet, R. (1981). Mechanics of Acetabular Fractures. In: Fractures of the Acetabulum. Springer-Verlag, New York. pp. 7-12.
- Afoke, N. Y. P, Byers, P. D. and Hutton, W. C. (1987). Contact pressures in the human hip joint. Journal of Bone and Joint Surgery., 69B(4), 536-541.
- Bay, B. K., Hamel, A. J., Olson, S. A., Sharkey, N. A. (1996). Statically equivalent load and support conditions produce different hip joint contact pressures and periacetabular strains. Journal of Biomechanics, 33 (2). 193-196.
- Von Eisenhart-Rothe, R., Eckstein, F., Landgraf, J. et al. (1996). Direct comparison of contact areas, contact stress and subchondral mineralization in human hip joint specimens. Anatomy and Embryology, 195. 279-288.
- Konrath, G. A., Hamel, A. J., Sharkey, N. A., Bay, B. K., Olson, S. A., (1998). Biomechanical evaluation of a low anterior wall fracture: correlation with the CT subchondral arc. Journal of Orthopedic Trauma 12(3), 152-158.