Lauri Connelly, Yicheng Jia, Maria L. Toro, Mary Ellen Stoykov, Robert V. Kenyon, Member IEEE, and Derek G. Kamper, Member, IEEE
Abstract
While a number of devices have recently been developed to facilitate hand rehabilitation after stroke, most place some restrictions on movement of the digits or arm. Thus, a novel glove was developed which can provide independent extension assistance to each digit while still allowing full arm movement. This pneumatic glove, the PneuGlove, can be used for training grasp-and-release movements either with real objects or with virtual objects in a virtual reality environment. Two groups of stroke survivors, with 7 subjects in each group, completed a 6-week rehabilitation training protocol, consisting of three one-hour sessions held each week. One group wore the PneuGlove during training, performed both within a novel virtual reality environment and outside of it with physical objects, while the other group completed the same training without the device. Across subjects, significant improvements were observed in the Fugl-Meyer Assessment for the upper extremity (p<0.001), the hand/wrist portion of the Fugl-Meyer Assessment (p<0.001), the Box and Blocks test (p<0.05), and palmar pinch strength (p<0.05). While changes in the two groups were not statistically different, the group using the PneuGlove did show greater mean improvement on each of these measures, such as gains of 3.6 vs. 2.4 points on the hand/wrist portion of the Fugl-Meyer Assessment and 14 N vs. 5 N in palmar pinch.
I. INTRODUCTION
Stroke is a leading cause of disability. Approximately 780,000 Americans suffer a new or recurring stroke each year with 30-60% of these individuals subsequently reporting arm impairments six months poststroke [1]. In 1998, more than 1,100,000 Americans reported post-stroke functional limitations [2]. Post-stroke upper extremity hemiparesis, including weakness of the contralesional arm and hand, is one of the most common conditions addressed by occupational therapists and physical therapists. In fact, finger extension is the most common motor deficit.
Therapeutic techniques are needed to address the problem of hemiparesis of the hand. An evidence-based review suggests that rehabilitation interventions which incorporate intensive training of active repetitive movements might increase upper extremity function after stroke [3]. This finding corroborates conclusions from studies examining animal models of stroke that motor practice should be intense, repetitive, and skill based [4]. One such technique which has been described at length in the literature is constraint-induced movement therapy, in which intensive focus is placed on use of the affected limb [5, 6]. As impairment after stroke may severely limit task performance, however, mechatronic devices have been developed to facilitate repetitive practice.
The use of robots in therapy has shown promise, especially for the proximal arm [7-12]. Initial results for the forearm and wrist have also been encouraging [13-15]. Experience with the hand has been more limited, although a number of mechatronic devices have been developed which can actively assist movement of the digits [16-20]. Training with the Rutgers Master II-ND [21], Hand Mentor [22], and HWARD [15], for example, has shown promise in decreasing impairment in rehabilitation trials. Unfortunately, few devices permit free movement of the arm, complete range of motion of the digits, and control of individual digits, all of which may be beneficial to rehabilitative training. While the Amadeo System (Tyromotion GmbH, Graz, Austria), for example, does provide independent control of the digits, hand location and orientation are fixed.
A common difficulty with using robotics or other mechatronic devices is the confinement of movement dictated by the particular system. Restrictions in device portability may limit the engagement and motivation of the user. Use of virtual reality (VR) has emerged in an effort to promote task oriented and repetitive movement training of new motor skills while using a variety of stimulating environments [23].
Positive results have been reported following the use of virtual reality for retraining upper extremity hemiparesis [21, 23-29]. For example, Adamovich et al. found decreased impairment and improved performance of real world activities following training of hand and finger function with a VR system [30]. Other possible benefits of VR include rapid transition between tasks and unlimited affordances [23], stimulation of cognitive networks [31], and increased activation in secondary motor areas [31].
Thus, the goal of this study was to develop and test a pneumatically actuated glove, the PneuGlove, for use in therapeutic training of hand movement after stroke. The PneuGlove was designed to take advantage of the residual control of finger flexion that we have observed in stroke survivors, even those with severe hand impairment [32, 33], and, thus, to assist only digit extension. Proper opening of the hand is essential to functional use of the hand. Indeed, successful completion of grasp-and-release, one of the fundamental functional tasks performed with the hand, requires execution of a series of subtasks, including properly opening and positioning the hand, gripping the object, and then letting go of the object. While difficulties with producing proper gripping forces have been described [34, 35], extending the digits to position them for grasping and then opening the hand for object release tend to be the most challenging subcomponents [32, 36]. The PneuGlove can assist digit extension as needed in order to facilitate training of hand movements. As VR environments may help to promote practice of hand motions, the PneuGlove was also designed to be compatible with a fully immersive VR environment, developed in conjunction in our laboratory. A pilot study was conducted to assess efficacy of the system in stroke survivors with chronic hand hemiparesis.
II.DEVELOPMENT OF TRAINING ENVIRONMENT
A novel externally actuated glove, the PneuGlove [37, 38], was developed to provide extension assistance to individual digits. The glove can be used in conjunction with a wireless system, the Shadow Monitor [39, 40], which measures joint kinematics using bend sensors (Flexpoint Sensors, Draper, UT). The PneuGlove can be used either with real objects or within a novel immersive VR environment developed expressly for promoting hand rehabilitation.
A. PneuGlove
The PneuGlove is a novel glove which utilizes air pressure to provide assistance of digit extension in order to promote practice of hand movements, such as grasp-andrelease tasks. Extension of the fingers and thumb is essential for positioning the digits for grasp and for enabling release of the object during the gr
asp-and-release task. The PneuGlove consists of a custom-fabricated air bladder (Vinyl Technology, Inc., Monrovia, CA) on the palmar side of the glove and a lycra backing on the dorsal side. A zipper sewn into the lycra aids in donning and doffing the glove, especially for hands with flexor hypertonia (see Fig. 1b). Also, on the dorsal side of the glove, 10 polyester sleeve pockets are sewn at locations corresponding to the metacarpophalangeal (MCP) and proximal interphalangeal (PIP) joints of the fingers and the MCP and interphalangeal (IP) joints of the thumb to hold the flexion sensors from the Shadow Monitor.
The custom air bladder, fabricated from polyurethane, has a series of 5 independent channels, one for each digit (Fig. 1). Air pressure within a channel creates an extension force which pushes the digit further into extension. Open-cell foam within each channel helps to reduce restriction of air flow within the channel during hand flexion. Each bladder channel is physically isolated with respect to the others so that assistance of each digit is achieved independently. This manner of extension assistance precludes potential problems with joint subluxation and hyperextension. When the air pressure is removed, the digit is free to flex (minimal impedance is offered by the deflated glove). Thus, it is possible to grab real objects with the PneuGlove.
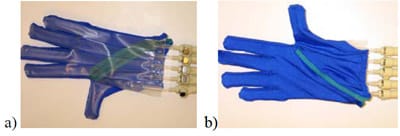
Fig. 1. Picture of the PneuGlove. a) View from the palmar side of the glove. Polyurethane chambers contact the palmar side of the digits. b) View from the dorsal side. A lycra backing contacts the dorsal side of the hand. The zipper allows for easier donning and doffing.
Each channel is connected, through air valves and flexible tubing, to an electropneumatic servo valve (QB02005, Proportion-Air, McCordsville, IN). The servo valve provides air pressure between 0 – 10 psi, linearly proportional to a command voltage. A software program written in Visual Basic (Microsoft Visual Studio, Microsoft Corp., Seattle, WA) controls the PneuGlove through a personal computer.
B. Final Stage
The PneuGlove is well suited to be used in conjunction with a VR environment, as it can provide a measure of haptic feedback in addition to assistance of finger extension. Our VR application uses the Wide5 Head Mounted Display (HMD) as the display device (Fakespace Labs, Inc., Mountain View, CA, Mechdyne). The Wide5 HMD provides immersive stereo vision and has a very wide field-of-view (FOV): 140° in the vertical direction and 150° in the horizontal direction. The wide FOV allows the user to move her/his eyes rather than head to view different parts of the environment, which is important when working with stroke survivors, as neck control can be compromised, especially when lifting the arm [38].
The virtual scene for this study was created through the coordination of several software packages. The Coin3D system (Systems In Motion, Oslo, Norway) implements the scene graphs, the CAVELib™ software (Mechdyne, Marshaltown, IA) controls scene display, and the trackd® tool (VRCO) reads in head postion and orientation data and provides them to the rendering thread transparently. The entire scene is updated at 20 Hz in accordance with head position which is continuously monitored using a magnetic tracker (Flock of Birds, Ascension Tech, Burlington, VT) mounted to the HMD.
The virtual scene developed for the current project consists of a room with 4 walls, a floor, and a ceiling (see Fig. 2a). Texturing and a table in the room provide cues to depth. The user is able to see her/his virtual hand in the scene, as well as virtual objects and the training context. A set of virtual everyday objects, such as tennis balls, soccer balls, glasses, and mugs, of different radii (thereby requiring different amounts of digit extension in order to sufficiently open the hand for grasp) were created and can be randomly accessed to provide a different object for each trial. During a trial, the user attempts to perform grasp-andrelease of the presented object within an allotted time.
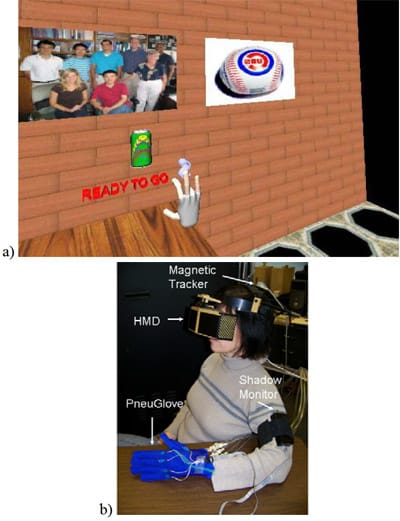
Fig. 2. a) Two-dimensional view of the virtual environment seen by the user. Entire room (floor, ceiling, and all 4 walls) is visible. Virtual hand is directly controlled by user’s hand. User attempts grasp and release the object displayed for each trial (e.g., the soda can). b) View of actual system. HMD displays virtual environment in stereo; magnetic tracker measures head position and orientation for updating scene; Shadow Monitor records joint angles for the digits for controlling the virtual hand; and PneuGlove provides extension assistance.
The PneuGlove and VR can either be run independently or in an integrated manner. Each is controlled by a separate computer, which communicates with its counterpart using TCP/IP protocol. The PneuGlove is controlled by the Visual Basic program which receives angle input from the Shadow Monitor and provides output to the electropneumatic servo valves. Assistance for digit extension may be given during the hand opening and release stages of the grasp-and-release task. When used in conjunction with the VR and virtual objects, the PneuGlove is programmed to operate in an assist-as-needed mode in which assistance to digit extension is provided only if actual joint angles fail to follow the desired trajectories, which are determined by the dimensions of the virtual object. PID feedback control is implemented, with a dependence upon the sign of the error signal (no assistance is provided if the actual extension angle exceeds the desired extension angle). The Visual Basic program also transmits the joint angle data to the VR computer for use in updating the virtual hand in the VR scene. Additionally, the program specifies which objects are to appear in the scenes and the dimensions of these objects. It computes the desired joint angles needed to shape the hand for grasp, based on the dimensions of the objects and the size of the user’s hand, and transmits these desired angles to the VR
The VR computer tracks head movement (and arm movement, if desired for training of reach-to-grasp) and generates the necessary images to send to the HMD, thereby updating the view in correspondence with head movement and the digit joint angles. The Visual C++ program on the VR computer also determines success or failure of hand opening for grasp of the virtual object, as based on the actual and desired joint angle data. A trial is deemed successful if the hand opens sufficiently to permit grasp of the object. If the grasp is successful and the PneuGlove is being used, the PneuGlove computer initiates a haptic mode in which the PneuGlove is used to simulate the virtual object by offering resistance against further flexion of the joints beyond the angles which define the surface of the object.
The Visual Basic program also transmits the joint angle data to the VR computer for use in updating the virtual hand in the VR scene. Additionally, the program specifies which objects are to appear in the scenes and the dimensions of these objects. It computes the desired joint angles needed to shape the hand for grasp, based on the dimensions of the objects and the size of the user’s hand, and transmits these desired angles to the VR
The VR computer tracks head movement (and arm movement, if desired for training of reach-to-grasp) and generates the necessary images to send to the HMD, thereby updating the view in correspondence with head movement and the digit joint angles. The Visual C++
program on the VR computer also determines success or failure of hand opening for grasp of the virtual object, as based on the actual and desired joint angle data. A trial is deemed successful if the hand opens sufficiently to permit grasp of the object. If the grasp is successful and the PneuGlove is being used, the PneuGlove computer initiates a haptic mode in which the PneuGlove is used to simulate the virtual object by offering resistance against further flexion of the joints beyond the angles which define the surface of the object.
III. EXPERIMENTAL DESIGN
A. Device Characterization
The amount of kinematic and kinetic assistance provided by the PneuGlove was characterized in a set of experiments. Resistance to flexion was measured during imposed rotation of the MCP joints of a neurologically intact volunteer who was wearing the PneuGlove. The MCP joints were rotated isokinetically by a servomotor (JR16M4CH-1, PMI Motion Technologies, Kollmorgen Co., Waltham, MA) from 10° of extension to 70° of MCP flexion at 10°/s, both with the glove deflated and with it inflated to 10 psi [41]. The resulting extension torque was recorded with a torque transducer.
Additionally, the isometric force generated at the index fingertip was measured with a 6 degree-of-freedom load cell (20E12A, JR3, Inc., Woodland, CA) in response to inflation of the corresponding air chamber for that digit to 10 psi. Finally, maximum opening speed of the glove was examined by measuring the speed of fingertip movement in response to inflation of the PneuGlove. Fingertip motion with respect to the MCP joint was optically tracked for each digit with an Optotrak camera system (Optotrak Certus®, Northern Digital Inc., Ontario, Canada).
A pilot study was also undertaken to evaluate the feasibility of utilizing this system in a rehabilitation paradigm. Subjects came to the laboratory for 18 training sessions, each consisting of a hybrid therapy approach in which 30 minutes of the session were used to practice grasp-and-release tasks in a VR environment and the other 30 minutes were devoted to hand therapy focused on graspand- release tasks with real objects.
Subjects
Subjects were recruited from the Rehabilitation Institute of Chicago Clinical Neuroscience Research Registry and from within the Rehabilitation Institute of Chicago. Eligible participants met the following criteria:
- at least 18 years of age;
- at least 6 months post stroke;
- at least 6 months post Botox injections in affected upper extremity;
- Stage 4 or 5 on the Chedoke-McMaster Stroke Assessment of the Hand Stage of Recovery;
- sufficient cognitive status to follow complex commands, including auditory commands without sight of commander
- full field of vision and hemispheric attention;
- the ability to tolerate 30 minutes of HMD wear.
Prospective subjects could not be enrolled in therapy during the duration of the study, including the follow-up evaluations. Sixteen stroke survivors, who met these criteria, participated in the study. Informed consent was obtained for each of the 16 subjects, 10 females and 6 males, in accordance with the requirements of the Institutional Review Board of Northwestern University.
Eight of the subjects were randomized to a group which used only the Shadow Glove during the VR training (“VR-only”) while the other group of 8 subjects additionally used the PneuGlove (“VR+Glove”). This group wore the PneuGlove during training with both virtual and real objects. For the 14 subjects (7 in each group) who completed the entire study, there were no significant differences in age or time (months post-stroke) between the groups. Baseline characteristics for these subjects are presented in Table I. All of these subjects were formerly right-hand dominant, with 7 having primary impairment of the left hand and 7 having primary impairment of the right hand. Twelve of the subjects were rated to be at Stage 4 on the Chedoke-McMaster Assessment for the hand, while two were rated to be at Stage 5 at baseline.
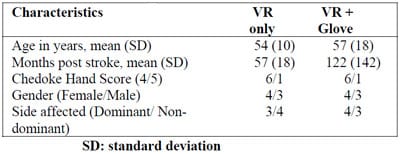
Table I Subject Characteristics
Training Protocol
Upon completion of evaluations, all subjects participated in treatment sessions three times per week for six weeks (total of 18 sessions) as members of either the VR+Glove group or the VR-only group. As tactile feedback and functional task training may be important for rehabilitation [42], both groups received approximately 30 minutes of training in the VR environment followed by 30 minutes of training in functional use of real objects during each treatment session.
All subjects used the Shadow Monitor in conjunction with the VR training. The five joint angle sensors were placed over either a PIP/IP joint or an MCP joint for each digit. The therapist selected the joints to be monitored based on which joints most needed improvement in active range of motion to complete functional activities.
Thorough sensor calibration for each subject was performed on the first day of training and these calibration curves were used on subsequent days, unless they needed to be updated. Set-up time for the Shadow Glove and the VR was approximately 5 minutes, exclusive of any time needed for calibration.
In the VR environment, 60 grasp-and-release trials were performed for each session. For each trial, the subject was instructed to sufficiently extend her/his fingers to position the hand for grasp of the object shown. If the subject was successful, the virtual object was grasped by the virtual hand. If not, the virtual object fell to the ground; the digits of the virtual hand which failed to open sufficiently were shown in red. Auditory feedback of success or failure was additionally provided and a running score, visible to the subject in the virtual scene, was maintained. After a successful hand opening phase, the subject was cued to grasp the virtual object, and then cued to open the hand and release the object. The types of virtual objects presented during a session could be selected by the therapist to make the task more challenging (larger objects) or less challenging (smaller objects) as needed.
The VR+Glove group additionally wore the PneuGlove. As size of the virtual objects was known, the degree of assistance provided by the PneuGlove was determined according to the computer algorithm described previously. Peak assistance was limited such that passive wearing of the PneuGlove did not ensure success. The VR+Glove group also received haptic feedback during the hold phase in that the glove provided resistance to digit flexion beyond the boundaries of the virtual object. For object release, the PneuGlove was slightly deflated prior to the signal to release the object, in order to allow the subject time to actively extend their fingers before air assistance was provided.
Outside of the virtual environment, a task-oriented approach to training was employed in conjunction with real objects for another 30-minute training period. Subjects were afforded the opportunity to choose between activities they felt best addressed real-life performance problems, such as grasping and releasing dishware to set a table or opening a medication bottle and picking up pills to set up a weekly pillbox. Additionally, subjects were encouraged and guided to analyze their own movement during activities to promote active problem solving and to generalize to their home setting. Movement practice focused on grasp-andrelease, pinch-and-release, digit individuation, and finger extension. In addition, wrist, elbow and shoulder movements were addressed as appropriate during reach-tograsp activities. Tasks could be performed while standing, sitting, or walking.
For the VR+Glove group in the real environment, the level and timing of pneumatic assistance were directly controlled by the therapist. Once the desired hand opening was attained, air pressure in the glove could be released to permit grasping of the object or movement of the digit. The therapist could also specify to which digit or combination of digits the assistance was provided. Pneumatic resistance to digit flexion was introduced on occasion to promote strengthening of grasp or pinch.
Evaluation
Evaluations were conducted at pre-intervention, postintervention, and at follow-up (four weeks postintervention) by a research occupational therapist. Testing duration, including breaks, lasted approximately 1.5 hours for each evaluation session. Outcome measures included the Fugl-Meyer Assessment of Upper Extremity Function (FMUE) [43], the hand/wrist portion of the FMUE, the Box and Blocks test [44], and measurements of grip strength, lateral pinch, and palmar pinch strength. The FMUE is a test of post-stroke upper extremity function and impairment. The Box and Blocks test is a measure of gross grasp-and-release function. Grip strength, lateral pinch strength, and palmar pinch strength assess not only peak muscle force generation, but also the ability to properly apply hand forces.
Statistical Analysis
The effect of training on the outcome measures was analyzed using multivariate analysis of variance (MANOVA). The within-subject factor was session (baseline, post-training, and follow-up) and the betweensubject factor was group (VR-only and VR+Glove). The outcome measures were the FMUE, the Box and Blocks, and the strength tests. For independent variables which demonstrated a significant influence, post-hoc univariate analyses were conducted for each dependent variable.
IV. RESULTS
A. Glove Characterization
Resistance provided by the PneuGlove to imposed flexion of the MCP joints was assessed in a neurologically intact user. The difference in the recorded MCP extension torque between when the PneuGlove was inflated and deflated revealed, as expected, a dependence upon joint angle (see Fig. 3). By design, the glove exerts little force against the digits when the digits are fully extended (0° of flexion). The extension torque produced by the glove about the MCP joints reached a peak of 2.7 N-m at 70° of flexion. Testing of the isometric forces generated at the index fingertip of the index finger was also performed. Inflation of the glove resulted in 3.0 ± 0.3 N of extension force at the fingertip when the MCP joint was flexed 60°.
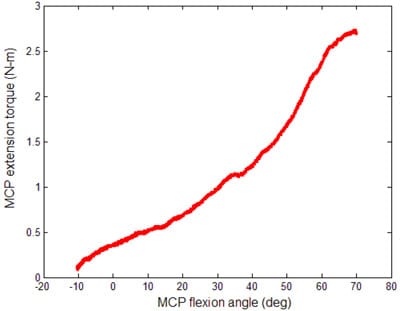
Fig. 3. Extension MCP resistance torque provided by PneuGlove during imposed flexion rotation of MCP joints. Curve shows mean values across three trials.
Maximum speed of opening was assessed in a relaxed, neurologically intact subject wearing the PneuGlove. Peak speed of the fingertip with respect to the MCP joint typically surpassed 10 cm/s. Average peak speed for the index finger, for example, was 12 cm/s. The trajectory of the fingertip followed a natural curvilinear path with a largely unimodal speed profile, despite no explicit control of position or speed (see Fig. 4).
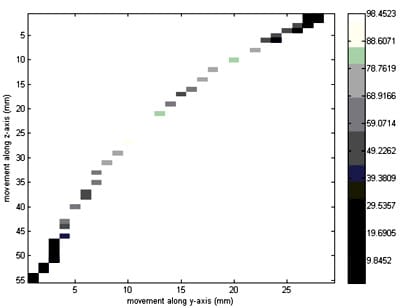
Fig. 4. Sample trial showing planar motion of the index fingertip produced by inflation of the PneuGlove to 10 psi. Instantaneous speed at each point along trajectory is encoded by color (lighter color indicates higher speed, in mm/s).
B. Pilot Study
For the pilot study, all subjects tolerated the training, including the immersive VR, well. One subject from each group was forced to withdraw due to issues related to work and to moving away from the area, but neither did so from dissatisfaction with the study. Subjects in both groups completed 60 full hand grasp-and-release trials in the VR environment during each training session, while the VRonly group completed 119 (± standard error of 14) finger/hand movements in the real environment and the VR+Glove group completed 97 (± 4). There was no significant difference between the groups in the number of repetitions (p > 0.15).
The MANOVA examining training effects on the clinical measures showed an overall significant effect of session (F= 3.216, p=0.003), indicating that both groups improved due to the training. Univariate analysis revealed significant main effects of session for the following clinical measures: FMUE (F= 20.03, p< 0.001) (see Fig. 5); the hand/wrist section of the FMUE (F= 17.76, p< 0.001); the Box and Block test (F=7.2, p=0.012); and palmar pinch (F=5.1, p=0.038). For all of these measures, both the postintervention and the follow-up mean scores were higher than the baseline score. There were no significant effects of session for lateral pinch (F=, 1.0, p=0.37) or grip strength (F= 2.98, p=0.074), indicating no substantial improvement in either measure as a result of the training.
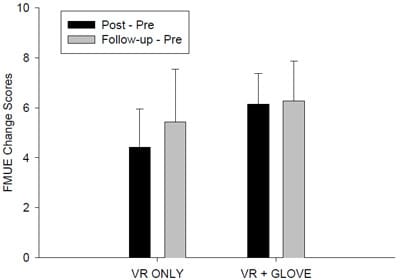
Fig. 5. Group mean change scores and standard error bars for Fugl-Meyer Upper Extremity evaluation (FMUE). VR ONLY group did not use the PneuGlove for training while the VR + GLOVE group did. Changes in scores from baseline to the completion of training (Post-Pre, darker bars) and from baseline to the follow-up evaluation (Follow-up – Pre, lighter bars) are shown.
For the VR+Glove group, mean improvement in the FMUE was 6.1 points (± 1.2) post-intervention and 6.3 (± 1.6) points at the one-month follow-up (66-point scale). The majority of that improvement was in the hand/wrist section of the FMUE (3.9 ± 0.7 points at post-intervention and 3.7 ± 0.4 points at the follow-up).
Table II presents the mean pre-intervention, postintervention, and one month follow-up scores by group. There were no overall significant differences between the groups (F=0.946, p=0.904), and there was no session by group interaction for any of the dependent measures. However, the mean change scores from baseline to immediately after treatment (Post – Pre) and from baseline to the one-month follow-up (Follow-up – Pre) were greater
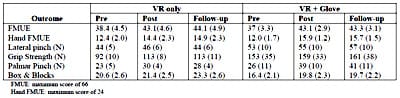
Table II OUTCOME MEASURES BY GROUP: MEAN (STANDARD ERROR)
in the VR+Glove group than in the VR-only group for each of the dependent variables which exhibited a significant session effect. The mean force (± standard error) improvement in palmar pinch, for example, was 12 (± 6) N for the VR+Glove group as compared to 7 (± 4) N for the VR-only group. This difference widened at the one-month follow-up as the VR+Glove group improved by 14 (± 8) N from baseline as compared to 5 (± 4) N for the VR-only group (see Fig. 6). For the hand/wrist portion of the FMUE, the VR+Glove group increased their average score from pre-intervention to post-intervention by 3.9 (± 0.7) points while the VR only group improved by 2.0 (± 1.0) points. At follow-up, the changes from baseline were 3.7 (± 0.4) for the VR+Glove group and 2.4 (± 1.2) from the VR only group. Gains in this measure for the group using the PneuGlove were much more consistent (Fig. 7). Thus, post-hoc paired t-tests for this group showed significant change for both Post-Pre (p< 0.002) and Foll
ow-up – Pre (p< 0.001). For the group training without the PneuGlove, these t-test results were not significant (Post-Pre: p=0.10 and Follw-up – Pre, p=0.09).
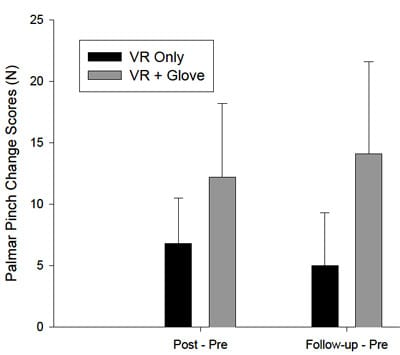
Fig. 6. Group mean change scores and standard errors for palmar pinch strength. Darker bars: VR-only group; Lighter bars: VR+Glove group.
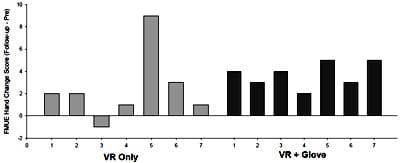
Fig. 7. Change scores (Follow-up – Pre-intervention) for each subject for the hand/wrist portion of the FMUE. While 6 of the 7 subjects in the VR + Glove group had at least a 3-point gain in this score (equivalent to more than 10% of the full scale) only 2 of the 7 subjects in the VR-only group attained this level of improvement.
V.DISCUSSION
The PneuGlove was designed to assist digit extension during the hand opening phase of grasp and during object release. Our testing showed that the PneuGlove exerted an extension torque of up to 2.7 N-m across the MCP joints of the four fingers, when fully inflated to 10 psi, in response to imposed MCP rotation. This magnitude is greater than the flexion torque resulting from the typical spastic stretch reflex in stroke survivors, even with severe hand impairment [41] and is on a par with the involuntary flexion torque that may result due to excessive flexor coactivation during attempted voluntary extension in stroke survivors [45]. With the air evacuated, the PneuGlove is still very compliant, such that there is minimal interference with closing the hand completely. Due to the distribution of the pressure along the digit, the glove is quite comfortable to use and there are no concerns about joint hyperextension or subluxation.
In a relaxed hand, fingertip speed during imposed hand opening can exceed 10 cm/s. While this is considerably less than what can be actively achieved in an unimpaired hand, it is sufficient for training. The speed and force of opening could be improved with greater pressure, but at the potential expense of reduced compliance of the glove (the sturdier air bladders required might passively inhibit hand closing).
In occupational therapy sessions, the focus on digit extension is practical and desirable for work on improving functional ability. As the PneuGlove is lightweight and permits a large workspace, it affords great flexibility for treatment. The user can sit, stand, or even move about while wearing the device. The therapist can direct the user to incorporate wrist, elbow and shoulder control, if appropriate, in conjunction with the hand movements. Training task difficulty can thus be controlled by the or subject position. Graphical feedback of assistance and range of motion from the device enables the therapist to gauge progress and make adjustments during a therapy session. The ability to control assistance/resistance to individual digits was also important as preferential impairment of specific digits was common among our subjects.
For the pilot study, improvement in a number of the outcome measures after completion of the training protocol was observed for both groups of subjects. Significant increases in the upper extremity Fugl-Meyer Assessment scores were achieved. The change of 6 points, or almost 10% of the total scale, is considerable for rehabilitation of chronic impairment and compares quite favorably to a recent robotics study for upper extremity hemiparesis.[9] Importantly, there was also a significant improvement in the hand/wrist portion of the Fugl-Meyer scale. Thus, this targeted hand intervention did, indeed, have a beneficial effect on the hand. This improvement on the impairment scale translated to improved task performance, as evidenced by the Box and Blocks test, and palmar pinch. Increases in palmar pinch strength, without increases in grip or lateral pinch strength, most likely resulted from improved coordination and applied force direction between the thumb and index finger. It has been shown that force direction during grasp is compromised following stroke.[32]
In accordance with the findings of others [21, 23-30, 46, 47], we observed that training in virtual reality environments may be beneficial for stroke survivors. Despite the simplicity of the current VR environment, subjects were enthusiastic about using it. They found the challenge of a scored task and the visual feedback displaying which digit(s) had failed to open sufficiently to be especially motivating. Subjects reported feeling mentally and physically challenged during each VR session, but productively so. Therapists felt that more whole hand grasp-and-release trials in a given time period could be performed with the virtual, as opposed to real, objects. Although the VR was fully immersive, there were no reported incidents of motion sickness or vertigo. Two drawbacks were that while the HMD used did permit a very wide field-of-view, it was fairly heavy (although the weight was partially offset by a counterbalance system) and had relatively low resolution.
cWhile there was no statistically significant difference in the changes across the dependent variables for the two groups, (one group using the PneuGlove and the other not), the mean change scores for all of the dependent variables were greater for the subjects using the PneuGlove. Immediately following completion of training, the mean improvements in the hand section of the FMUE, palmar pinch, and Box and Blocks test were twice as large for the group using the PneuGlove as compared to the group without it. While it should be noted that the group using the PneuGlove did receive some strength training in flexing against the glove that the other group did not, there were no significant changes in either grip or lateral pinch strength for either group.
For this study, the PneuGlove was used to institute an assist-as-needed algorithm. Other algorithms, such as error-amplification and performance-based adaptation (see [48] for a review) may prove more efficacious. As the PneuGlove cannot independently control joints within a digit, however, the ability to precisely impose some of the more complex algorithms may be limited. Additionally, practice of other grasps, such as lateral or palmar pinch, are more difficult to facilitate with the PneuGlove as the user must help to properly position the hand.
In future applications, we believe the PneuGlove could be especially useful for training finger individuation. One research group recently developed a system in which a virtual piano keyboard is integrated with the CyberGrasp device (VRLOGIC GmbH, Dieburg, Germany) to promote rehabilitation of finger individuation [49]. We believe our system could be used similarly. Toward that end, we are developing a more engaging VR environment which would incorporate these types of movements, along with the grasp-and-release tasks. Further adjustment of the air bladders at the ends of the glove would also be beneficial to reduce interference with fine pincer grasp activities when using real objects.
ACKNOWLEDGMENT
The authors wish to thank Dr. Xun Luo and Ms. Tiffany Kline for their efforts in helping to develop the PneuGlove and the VR environment and Ms. Bridget Iwamuro for her assistance with subject training.
REFERENCES
- G. Kwakkel, B. J. Kollen, J. van der Grond, and A. J. Prevo, “Probability of regaining dexterity in the flaccid upper limb: impact of severity of paresis and time since onset in acute stroke,” Stroke, vol. 34, pp. 2181-6, Sep 2003.
- W. Rosamond, K. Flegal, K. Furie, A. Go, K
. Greenlund, N. Haase, S. M. Hailpern, M. Ho, V. Howard, B. Kissela, S. Kittner, D. Lloyd-Jones, M. McDermott, J. Meigs, C. Moy, G. Nichol, C. O’Donnell, V. Roger, P. Sorlie, J. Steinberger, T. Thom, M. Wilson, and Y. Hong, “Heart disease and stroke statistics–2008 update: a report from the American Heart Association Statistics Committee and Stroke Statistics Subcommittee,” Circulation, vol. 117, pp. e25-146, Jan 29 2008. - vol. 117, pp. e25-146, Jan 29 2008. R. P. Van Peppen, G. Kwakkel, S. Wood-Dauphinee, H. J. Hendriks, P. J. Van der Wees, and J. Dekker, “The impact of physical therapy on functional outcomes after stroke: what’s the evidence?,” Clin Rehabil, vol. 18, pp. 833-62, Dec 2004.
- E. J. Plautz, G. W. Milliken, and R. J. Nudo, “Effects of repetitive motor training on movement representations in adult squirrel monkeys: role of use versus learning,” Neurobiol Learn Mem, vol. 74, pp. 27-55, Jul 2000.
- S. L. Wolf, C. J. Winstein, J. P. Miller, E. Taub, G. Uswatte, D. Morris, C. Giuliani, K. E. Light, and D. Nichols-Larsen, “Effect of constraint-induced movement therapy on upper extremity function 3 to 9 months after stroke: the EXCITE randomized clinical trial,” Jama, vol. 296, pp. 2095-104, Nov 1 2006.
- S. L. Wolf, C. J. Winstein, J. P. Miller, P. A. Thompson, E. Taub, G. Uswatte, D. Morris, S. Blanton, D. Nichols-Larsen, and P. C. Clark, “Retention of upper limb function in stroke survivors who have received constraint-induced movement therapy: the EXCITE randomised trial,” Lancet Neurol, vol. 7, pp. 33-40, Jan 2008.
- M. Ferraro, J. J. Palazzolo, J. Krol, H. I. Krebs, N. Hogan, and B. T. Volpe, “Robot-aided sensorimotor arm training improves outcome in patients with chronic stroke,” Neurology, vol. 61, pp. 1604-7, Dec 9 2003.
- B. T. Volpe, H. I. Krebs, N. Hogan, L. Edelsteinn, C. M. Diels, and M. L. Aisen, “Robot training enhanced motor outcome in patients with stroke maintained over 3 years,” Neurology, vol. 53, pp. 1874-6, Nov 10 1999.
- S. J. Housman, K. M. Scott, and D. J. Reinkensmeyer, “A randomized controlled trial of gravity-supported, computer-enhanced arm exercise for individuals with severe hemiparesis,” Neurorehabil Neural Repair, vol. 23, pp. 505-14, Jun 2009.
- S. Coote, B. Murphy, W. Harwin, and E. Stokes, “The effect of the GENTLE/s robot-mediated therapy system on arm function after stroke,” Clin Rehabil, vol. 22, pp. 395-405, May 2008.
- S. Masiero, A. Celia, G. Rosati, and M. Armani, “Robotic-assisted rehabilitation of the upper limb after acute stroke,” Arch Phys Med Rehabil, vol. 88, pp. 142-9, Feb 2007.
- P. S. Lum, C. G. Burgar, P. C. Shor, M. Majmundar, and M. Van der Loos, “Robot-assisted movement training compared with conventional therapy techniques for the rehabilitation of upper-limb motor function after stroke,” Arch Phys Med Rehabil, vol. 83, pp. 952-9, Jul 2002.
- S. Hesse, C. Werner, M. Pohl, S. Rueckriem, J. Mehrholz, and M. L. Lingnau, “Computerized arm training improves the motor control of the severely affected arm after stroke: a single-blinded randomized trial in two centers,” Stroke, vol. 36, pp. 1960-6, Sep 2005.
- H. I. Krebs, S. Mernoff, S. E. Fasoli, R. Hughes, J. Stein, and N. Hogan, “A comparison of functional and impairment-based robotic training in severe to moderate chronic stroke: a pilot study,” NeuroRehabilitation, vol. 23, pp. 81-7, 2008.
- C. D. Takahashi, L. Der-Yeghiaian, V. Le, R. R. Motiwala, and S. C. Cramer, “Robot-based hand motor therapy after stroke,” Brain, vol. 131, pp. 425- 37, Feb 2008.
- L. Rosenstein, A. L. Ridgel, A. Thota, B. Samame, and J. L. Alberts, “Effects of combined robotic therapy and repetitive-task practice on upperextremity function in a patient with chronic stroke,” Am J Occup Ther, vol. 62, pp. 28-35, Jan-Feb 2008.
- A. Wege and A. Zimmermann, “Electromyography sensor based control for a hand exoskeleton,” in IEEE Interntational Conference on Robotics and Biomimetics, Sanya, China, 2007, pp. 1470-1475.
- S. Hesse, H. Kuhlmann, J. Wilk, C. Tomelleri, and S. G. Kirker, “A new electromechanical trainer for sensorimotor rehabilitation of paralysed fingers: a case series in chronic and acute stroke patients,” J Neuroeng Rehabil, vol. 5, p. 21, 2008.
- L. Dovat, O. Lambercy, R. Gassert, T. Maeder, T. Milner, T. C. Leong, and E. Burdet, “HandCARE: a cable-actuated rehabilitation system to train hand function after stroke,” IEEE Trans Neural Syst Rehabil Eng, vol. 16, pp. 582-91, Dec 2008.
- O. Lambercy, L. Dovat, R. Gassert, E. Burdet, C. L. Teo, and T. Milner, “A haptic knob for rehabilitation of hand function,” IEEE Trans Neural Syst Rehabil Eng, vol. 15, pp. 356-66, Sep 2007.
- D. Jack, R. Boian, A. S. Merians, M. Tremaine, G. C. Burdea, S. V. Adamovich, M. Recce, and H. Poizner, “Virtual reality-enhanced stroke rehabilitation,” IEEE Trans Neural Syst Rehabil Eng, vol. 9, pp. 308-18, Sep 2001.
- I. Sarakoglou, N. G. Tsagarakis, and D. G. Caldwell, “Occupational and physical therapy using a hand exoskeleton based exerciser,” in IEEE/RSJ International Conference on Intelligent Robots and Systems, Sendai, Japan, 2004, pp. 2973-2978.
- J. C. Stewart, S. C. Yeh, Y. Jung, H. Yoon, M. Whitford, S. Y. Chen, L. Li, M. McLaughlin, A. Rizzo, and C. J. Winstein, “Intervention to enhance skilled arm and hand movements after stroke: A feasibility study using a new virtual reality system,” J Neuroeng Rehabil, vol. 4, p. 21, 2007.
- S. V. Adamovich, A. S. Merians, R. Boian, M. Tremaine, G. S. Burdea, M. Recce, and H. Poizner, “A virtual reality based exercise system for hand rehabilitation post-stroke: transfer to function,” Conf Proc IEEE Eng Med Biol Soc, vol. 7, pp. 4936-9, 2004.
- J. Broeren, M. Rydmark, and K. S. Sunnerhagen, “Virtual reality and haptics as a training device for movement rehabilitation after stroke: a single-case study,” Arch Phys Med Rehabil, vol. 85, pp. 1247-50, Aug 2004.
- J. H. Crosbie, S. Lennon, M. D. McNeill, and S. M. McDonough, “Virtual reality in the rehabilitation of the upper limb after stroke: the user’s perspective,” Cyberpsychol Behav, vol. 9, pp. 137-41, Apr 2006.
- A. Henderson, N. Korner-Bitensky, and M. Levin, “Virtual reality in stroke rehabilitation: a systematic review of its effectiveness for upper limb motor recovery,” Top Stroke Rehabil, vol. 14, pp. 52-61, Mar-Apr 2007.
- Y. S. Lam, D. W. Man, S. F. Tam, and P. L. Weiss, “Virtual reality training for stroke rehabilitation,” NeuroRehabilitation, vol. 21, pp. 245-53, 2006.
- M. Rydmark, J. Broeren, and R. Pascher, “Stroke rehabilitation at home using virtual reality, haptics and telemedicine,” Stud Health Technol Inform, vol. 85, pp. 434-7, 2002.
- A. S. Merians, H. Poizner, R. Boian, G. Burdea, and S. Adamovich, “Sensorimotor training in a virtual reality environment: does it improve functional recovery poststroke?,” Neurorehabil Neural Repair, vol. 20, pp. 252-67, Jun 2006.
- K. August, J. A. Lewis, G. Chandar, A. Merians, B. Biswal, and S. Adamovich, “FMRI analysis of neural mechanisms underlying rehabilitation in virtual reality: activating secondary motor areas,” Conf Proc IEEE Eng Med Biol Soc, vol. 1, pp. 3692-5, 2006.
- E. G. Cruz, H. C. Waldinger, and D. G. Kamper, “Kinetic and kinematic workspaces of the index finger following stroke,” Brain, vol. 128, pp. 1112- 21, May 2005.
- D. G. Kamper, H. C. Fischer, E. G. Cruz, and W. Z. Rymer, “Weakness is the primary contributor to finger impairment in chronic stroke,” Arch Phys Med Rehabil, vol. 87, pp. 1262-9, Sep 2006.
- D. A. Nowak, C. Grefkes, M. Dafotakis, J. Kust, H. Karbe, and G. R. Fink, “Dexterity is impaired at both hands following unilateral subcortical middle cerebral artery stroke,” Eur J Neurosci, vol. 25, pp. 3173-84, May 2007.
- P. Raghav
an, J. W. Krakauer, and A. M. Gordon, “Impaired anticipatory control of fingertip forces in patients with a pure motor or sensorimotor lacunar syndrome,” Brain, vol. 129, pp. 1415-25, Jun 2006. - N. J. Seo, W. Z. Rymer, and D. G. Kamper, “Delays in grip initiation and termination in persons with stroke: effects of arm support and active muscle stretch exercise,” J Neurophysiol, vol. 101, pp. 3108- 15, Jun 2009.
- Y. Jia, M. Toro, X. Luo, S. Lau, R. Kenyon, and D. Kamper, “Integration of virtual reality and an assistive device for hand rehabilitation following stroke,” in IEEE/ICME International Conference & Exhibition on Complex Medical Engineering, Beijing, China, 2007.
- H. C. Fischer, K. Stubblefield, T. Kline, X. Luo, R. V. Kenyon, and D. G. Kamper, “Hand rehabilitation following stroke: a pilot study of assisted finger extension training in a virtual environment,” Top Stroke Rehabil, vol. 14, pp. 1-12, Jan-Feb 2007.
- L. K. Simone, N. Sundarrajan, X. Luo, Y. Jia, and D. G. Kamper, “A low cost instrumented glove for extended monitoring and functional hand assessment,” J Neurosci Methods, vol. 160, pp. 335- 48, Mar 15 2007.
- R. Gentner and J. Classen, “Development and evaluation of a low-cost sensor glove for assessment of human finger movements in neurophysiological settings,” J Neurosci Methods, vol. 178, pp. 138-47, Mar 30 2009.
- D. G. Kamper and W. Z. Rymer, “Quantitative features of the stretch response of extrinsic finger muscles in hemiparetic stroke,” Muscle Nerve, vol. 23, pp. 954-61, Jun 2000.
- R. J. Nudo, K. M. Friel, and S. W. Delia, “Role of sensory deficits in motor impairments after injury to primary motor cortex,” Neuropharmacology, vol. 39, pp. 733-42, Mar 3 2000.
- A. R. Fugl-Meyer, L. Jaasko, I. Leyman, S. Olsson, and S. Steglind, “The post-stroke hemiplegic patient. 1. a method for evaluation of physical performance,” Scand J Rehabil Med, vol. 7, pp. 13-31, 1975.
- V. Mathiowetz, G. Volland, N. Kashman, and K. Weber, “Adult norms for the Box and Block Test of manual dexterity,” Am J Occup Ther, vol. 39, pp. 386-91, Jun 1985.
- D. G. Kamper and W. Z. Rymer, “Impairment of voluntary control of finger motion following stroke: role of inappropriate muscle coactivation,” Muscle Nerve, vol. 24, pp. 673-81, May 2001.
- J. Broeren, L. Claesson, D. Goude, M. Rydmark, and K. S. Sunnerhagen, “Virtual rehabilitation in an activity centre for community-dwelling persons with stroke. The possibilities of 3-dimensional computer games,” Cerebrovasc Dis, vol. 26, pp. 289-96, 2008.
- J. H. Crosbie, S. Lennon, J. R. Basford, and S. M. McDonough, “Virtual reality in stroke rehabilitation: still more virtual than real,” Disabil Rehabil, vol. 29, pp. 1139-46; discussion 1147-52, Jul 30 2007.
- L. Marchal-Crespo and D. J. Reinkensmeyer, “Review of control strategies for robotic movement training after neurologic injury,” J Neuroeng Rehabil, vol. 6, p. 20, 2009.
- S. V. Adamovich, G. G. Fluet, A. Mathai, Q. Qiu, J. Lewis, and A. S. Merians, “Design of a complex virtual reality simulation to train finger motion for persons with hemiparesis: a proof of concept study,” J Neuroeng Rehabil, vol. 6, p. 28, 2009.