L. McCann*, E. Ingham, Z. Jin and J. Fisher
Institute of Medical and Biological Engineering, School of Mechanical Engineering, University of Leeds, UK
Summary
Background: Total meniscectomy has been shown to cause early-onset arthritis in the underlying cartilage and bone in the knee joint, demonstrating that the meniscus plays an important protective role in the load carrying capacity. Relationships between friction and wear in synovial joints are complex due to the biphasic nature of articular cartilage and the time dependency of tribological responses. Determination of friction and wear in the whole natural joint in vitro or in vivo is technically difficult and the tribological effect of meniscectomy has not been previously studied in an articulating knee joint.
Objective: The aim of this study was to use a tribological simulation of the medial compartmental bovine knee, to investigate friction and wear, with and without the meniscus. We hypothesised that meniscectomy would lead to elevated contact stress and frictional coefficient across the joint.
Methods: Skeletally mature bovine medial compartmental knee joints were dissected and mounted in a pendulum friction simulator, which was used to apply physiologically relevant loading and motion. Wear was quantified using micro-MRI scans and surface profilometry.
Results: Knees tested with the intact meniscus showed no change in surface roughness and no detectable cartilage loss or deformation. However, increased contact stress and frictional coefficient upon removal of the meniscus, led to immediate surface fibrillation, biomechanical wear and permanent deformation of cartilage.
Conclusions: This study presents, for the first time, an in vitro model simulation system to investigate the tribological effects of meniscectomy and meniscus repair and regeneration. Crown Copyright ª 2009 Published by Elsevier Ltd on behalf of Osteoarthritis Research Society International. All rights reserved.
Key words: Articular cartilage, Meniscus, Meniscectomy, Friction, Wear, Contact stress, Knee biomechanics.
Introduction
Total meniscectomy has been shown to induce osteoarthritic changes in the underlying cartilage and bone in the natural knee joint1-3, indicating that the meniscus plays an important protective role in the load carrying capacity. Previous biomechanical studies have shown that the meniscus acts to make the knee joint more conforming or congruent, distributing the forces across the joint onto a larger area of articular cartilage hence reducing contact stress4-7. Studies have shown that at least fifty percent of the load across the joint is transmitted through the meniscus5, and removal of the meniscus has been shown to increase stress in the tibial cartilage and subchondral bone6. This elevation of stress is believed to contribute to subsequent osteoarthritic changes. Currently, damaged menisci are repaired if at all possible.
Recent fundamental studies of the tribology of articular cartilage have indicated that both the frictional force and degradation and wear are dependent on the loading period and contact stresses. The relationships are, however, complex due to the biphasic nature of articular cartilage and the time dependency of the tribological responses8-15. Articular cartilage (AC) is composed of a network of fine collagen fibrils, within which hydrophilic proteoglycan aggregate molecules are immobilised and restrained. Between 60 and 85 percent of the cartilage matrix is water16. The high water content, combined with the porosity and compliance of cartilage make it an exceptional bearing material. The solid matrix (collagen and proteoglycans) together with the water determine the biomechanical behaviour of AC. Under loading AC expels interstitial fluid into its unloaded areas and into the joint capsule. During this exudation process, the pressure differences produced in the fluid phase that cause the flow also carry varying amounts of the load, effectively reducing the load carriage and stresses in the solid matrix and the frictional forces between opposing surfaces are minimised. When the load is removed the tissue recovers the lost water, due to its inherent swelling pressure8,17-20.
The tribological response of the whole natural knee as a biomechanical system cannot be predicted from simple geometry8-15 cartilage specimen tests. Determination of friction and wear in the whole natural joint in vitro or in vivo is technically difficult and the tribological effect of meniscectomy has not been previously studied in an articulating knee joint.
It is well known that removal of the meniscus in the medial compartment of the knee leads to the elevation of the friction force and friction coefficient across the joint. This increased friction as well as increased contact stresses leads to degradation and wear of cartilage. However, there has been no direct, experimental evidence of this elevation of friction, contact stress and subsequent cartilage degeneration. An understanding of the mechanism of degradation of AC and the time course of degradation following meniscectomy is important in research and development of interventional therapies for damaged menisci whether they be direct biomechanical, surgical or biological interventions. The aim of this study was to investigate the tribological response (friction and wear) of the medial compartment of the natural knee joint, with and without the intact meniscus, under simplified physiological loads and motion representative of the walking cycle, and to study the effect of two different levels of loading. These tests were carried out using a pendulum friction simulator.
Materials and methods
A medial compartmental knee tribological simulation was used to investigate the friction and wear properties of the medial compartmental knee, both with and without the meniscus. The simulator (Simulation Solutions, Manchester, UK) was used to apply physiological loads and motions to bovine knee joints, whilst directly measuring the friction force.
Bovine medial femoral condyles, from animals aged between 18 and 24 months, were dissected between 24 and 48 h after slaughter, provided the cartilage was free from damage and appeared ‘‘normal’’ i.e., no brown or red tinges and firm to touch. The dissection and set-up procedures have been described in our previous study21. The femoral condyles were articulated against their corresponding medial tibial surfaces: (1) with the meniscus and its attachments removed to simulate a meniscectomy (ACvs- AC_meniscectomy), or (2) with the meniscus and its attachments intact to simulate the natural situation (AC-vs-AC þ meniscus).
The pendulum friction simulator is a single-station machine (Fig. 1); comprising of a fixed frame which consists of a fricti
on measuring carriage, sitting on two externally pressurised hydrostatic bearings and a loading frame. This consists of a motion arm to which the femoral head is attached. A piezoelectric transducer connected to the front of the friction carriage determines the frictional torque within the system, by measuring the forces transferred between the fixed frame and the carriage. Load and displacement are applied through the femoral head via the loading frame and motion arm respectively. All cartilage surfaces were immersed in lubricant, 25% (v/v) bovine serum in physiological saline, throughout the test.
The motion (flexioneextension) and loading profiles used for the test were derived from British Standards (BS 14243-3:2004) for knee gait profiles for simulators. The friction simulator was unable to run the full gait cycle of flexioneextension as the range was too great. This limitation within the machine meant that a maximum swing phase angle of 23° was used, rather than ~60° in full gait.
A dynamic load was used. The maximum peak load (PL) will be reported in this paper. The load acting through a bovine knee joint has been reported to be 190 kg (420 lbs)22, which is equal to a force of over 1800 N. In this medial compartmental simulation, the maximum peak load was reduced to 1,000 N (as 60% of the knee joint load acts through the medial compartment). Since it has been previously hypothesised that the contact stress is a cause of osteoarthritis the study was repeated at a lower level of dynamic loading, with a peak load of 250 N.
The first experiments performed were femoral condyles articulating against tibial surfaces and meniscus (AC-vs-AC þ meniscus) with a maximum peak load of 1,000 N, resulting in contact stresses of approximately 5 MPa, for 3600 cycles at 1 hertz. The same loading conditions were used for the condyles articulating against the tibial surface without the meniscus (AC-vs-AC_meniscectomy). Finally, a second set of experiments were conducted at the lower level of loading.
The peak contact stress between each bearing was measured using prescale Fuji film, which was placed between the bearing surfaces, while the friction simulator applied the required load as described above. Following testing, the Fuji film was placed in a Spectrodensitometer (from X-Rite), the density of the pink colour was taken and PointScan software was used to convert this density value to pressure. It should be noted that it was impossible to take these measurements during the test. These measurements were taken prior to the test, and so it must be acknowledged that these contact stresses may have been modified during the test, particularly where cartilage degradation took place.
Different types of cartilage wear were observed following friction testing. Some samples displayed no damage upon visual inspection. Others showed mild biomechanical degradation in the form of surface fibrillation and permanent displacement of matrix material was observed. The wear was characterised using contact profilometry and µMRI scans.
A stylus profilometer, Talysurf 5 model (Taylor Hobson UK), was used to characterise alterations in the surface topography of AC on the condylar and tibial surfaces as outlined in our previous study14. The surface roughness parameter considered was Ra, the arithmetic mean of departures of the roughness profile from the mean line.
A 9.4 Tesla Bruker Biospin 400 NMR (nuclear magnetic resonance) vertical magnet was used to scan the femoral cartilage specimen after the test. These specimens were too large to scan pre-friction testing and were truncated following the test to fit in the NMR imaging tube. The tibial specimens and menisci did not fit in the imaging tube and could not be scanned, so volumetric wear measurements were limited to the femoral condyles only. A multi-spin multi-echo sequence was used obtaining eight echoes and the echo time (TE) was 10.5 ms and the repetition time (TR) was 4843.1 ms. The 256 by 256 matrix and the 20 µm by 20 µm field of view, yielded an in-plane pixel size of 78 µm x µ8 mm with 1 mm slice thickness. A custom written MATLAB program was used to calculate wear volumes, if any, on a slice-by-slice basis. The ‘‘worn’’ region was highlighted by refitting the original surface curvature and depicting the wear scar. The number of voxels inside the worn region were obtained and the size of each voxel was known to be 6.08 103 mm3. This method was validated using a pycnometer to measure the volume of a removed piece of cartilage, MRI scans were taken of the bulk specimen and the Matlab program was used to measure the worn region left behind. There was excellent correlation between the two methods (R2 ¼ 0.9995). Furthermore, there was excellent correlation when an interperson study (R2 ¼ 0.9965) and two intra-person studies (R2 ¼ 0.9828 and R2 ¼ 0.9471) were carried out.
Specimens were left to recover in phosphate buffered saline solution after tribological tests for approximately 24 h then stored at -20 °C before taking the surface and volume measurements. The surface roughness and volume changes were considered to be permanent, non-recovered and were probably the result of wear (material loss) and permanent deformation of the matrix and loss of water. At present it is not possible to quantify the relative contributions to the volume change.
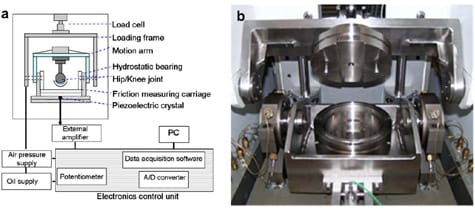
Fig. 1. Pendulum friction simulator: schematic representation of the friction simulator hardware.
Results
The variation of frictional coefficient as a function of loading time is shown in Fig. 2 for AC-vs-AC þ meniscus (the natural medial compartment) and AC-vs-AC_meniscectomy (meniscectomised medial compartment), at a maximum peak load of 1,000 N. For AC-vs-AC þ meniscus, peak contact stresses induced were 4.9 MPa. The friction coefficient ranged between 0.06 and 0.09. In contrast, the contact stresses were much higher without the meniscus, at 17.1 MPa and the friction coefficient ranged between 0.08 and 0.12. For the AC-vs-AC þ meniscus, there was no significant difference in the surface roughness measurements before and after the test and no detectable change in volume, indicating there was no detectable wear or detectable permanent deformation on the AC surfaces or meniscus after the experiments. For the meniscectomy specimens tested at the higher load, surface fibrillation of the articular surface was visible on all of those tested, as well as the displacement of some cartilage tissue.
All six specimens from the AC-vs-AC_meniscectomy group tested at this higher peak load showed similar wear scars. Using Talysurf to measure the surface roughness, it was found that the AC-vs-AC_meniscectomy specimens tested at this higher level of loading, increased in Ra from 1.0 µ before the test to 5.3 mm after and the tibial surfaces increased from 2.1 µ beforehand to 8.7 µm after. The change in volume was measured using the Bruker NMR scanner with an image resolution of 78 µm x 78 µm inplane pixel resolution (Fig. 3). The wear volume was found to be 71.15 ± 18.62 mm3 (n ¼ 6; mean ± 95% confidence intervals (CI)), using the custom written MATLAB program. The depth of the wear scar was found to be 44.9 ± 9.71% (n ¼ 6; mean ± 95% CI of the cartilage thickness. The specimens from the AC-vs-AC þ meniscus group, which showed no detectable volume change at this resolution, were scanned at higher resolution and it was found that there was no wear detectable to a resolution of 20 µm by 20 µm in-plane pixel resolution (Fig. 3).
The study was repeated at a lower level of dynamic loading, with a peak load of 250 N. The variation of friction coefficient as a function of loading time is shown in Fig. 4 for AC-vs-AC þ meniscus a
nd AC-vs-AC_meniscectomy, at a maximum peak load of 250 N.
For AC-vs-AC þ meniscus, contact stresses induced were 2.3 MPa and the friction coefficient ranged between 0.01 and 0.03. After the test there was no wear detectable to a resolution of 20 mm by 20 mm in-plane pixel size and no significant increase in surface roughness. For the meniscectomised bearing, the contact stress was 8.6 MPa, significantly higher (ANOVA P < 0.002) without the meniscus compared to the intact medial compartment. The friction coefficient ranged between 0.04 and 0.07 and there was no wear detectable at 20 mm by 20 mm in-plane pixel resolution (Fig. 3) and no significant change in surface roughness.
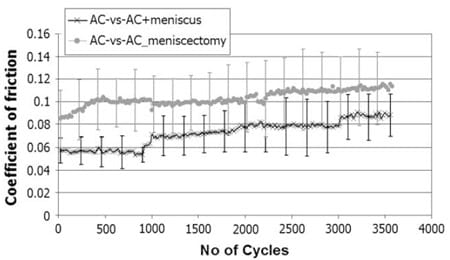
Fig. 2. Coefficient of friction over 3600 cycles for AC-vs-AC þ meniscus and AC-vs-AC_meniscectomy at a peak load of 1,000 N. Data presented as mean (n ¼ 6) ± 95% confidence intervals (CI). There was a statistical difference ( P < 0.05; ANOVA (Analysis of Variance) single factor þ T-Method) between the mean coefficients of friction (averaged over the 3,600 cycles).
The maximum average contact stress was measured at a range of loads (250 N, 500 N, 1,000 N and 1,500 N) with and without the meniscus and it was found to be significantly higher upon removal of the meniscus at all levels of loading (ANOVA P < 0.002). Even at 1,000 N loading, the contact stress for AC-vs-AC þ meniscus was only 4.9 MPa, which was lower than for the AC-vs-AC_meniscectomy at the lower level of loading of 250 N, where the contact stress was 8.6 MPa. On removal of the meniscus the congruity of the joint is decreased. The size of the contact areas is reduced and thus larger stresses are induced over smaller areas. The frictional shear stress (the product of the coefficient of friction and contact stress) was also examined for each experimental group. The relationship between friction shear stress and cartilage wear, determined from mMRI scans, is shown in Fig. 5. No wear was detected below friction shear stress levels of 0.5 MPa. However, the friction shear stress for the AC-vs-AC_meniscectomy was 1.77 MPa.
Discussion
It has been previously described that following meniscectomy the elevated contact stresses in the AC lead to long term biological changes in the AC and underlying bone and thus the early onset of osteoarthritis. Our studies have shown, for the first time experimentally, that removal of the meniscus leads to a direct elevation of friction, immediate surface fibrillation of the cartilage and direct biomechanical wear and permanent deformation of AC after less than 3600 cycles, 1-h of walking.
With the AC-vs-AC þ meniscus the peak contact stresses were less than 5 MPa, even at the higher level of loading, and the coefficient of friction was low. No wear was detected on the femoral condyles, menisci or tibial surfaces and there was no statistical difference between the surface roughness before and after testing, at either level of loading. This lack of wear is not surprising as the protective role of the meniscus is widely recognised1,3,23, as well as the joint congruency the meniscus provides and the resulting reduction in contact stress. The meniscus has been reported to absorb over 50% of the load acting through the joint5,7.
Despite the lack of (detectable) wear for the ACvs- AC þ meniscus bearing, when the level of loading and contact stress was artificially reduced (PL ¼ 250 N), the coefficient of friction was lower. It is possible that under higher physiological load and contact stress, that there was more solid-to-solid contact causing higher friction due to the biphasic nature of cartilage8. The same decrease in frictional coefficient, when the load and contact stress were reduced was found with the AC-vs-AC_meniscectomy bearing.
Without the meniscus, peak contact stresses rose to 8.6 MPa and 17.1 MPa, at the lower and higher level of loading, respectively. At a contact stress of 17.1 MPa, surface damage was visible on the femoral condyle surfaces, after less than 1-h testing. On removal of the meniscus the large joint loads pass through the cartilage surfaces. The congruity of the joint is decreased without the meniscus, and these larger loads must pass through smaller contacting areas. Furthermore, the meniscus is a biphasic material, without which the overall biphasic properties of the joint are compromised. The contact stress in this study was statistically higher for the meniscectomised bearing and the friction shear stress increased by three to four fold upon removal of the meniscus (Fig. 5). At a friction shear stress of 1.77 MPa, surface fibrillation was found on the femoral condyles. However, there was no detectable wear or degradation at the lower level of loading where the friction shear stress was 0.4 MPa. For the three bearings for which no wear was detected, the friction shear stress remained below 0.5 MPa. Therefore, although cartilage can withstand large compressive forces, due to the biphasic fluid load support, it may have low resistance to shear stresses at the surface. Based on this and the results of the current study, we hypothesise that the friction shear stress is a key factor in the degradation of articular cartilage. Further tests are required to determine the exact level of friction shear stress at which cartilage degradation begins, particularly for the meniscectomised bearing.
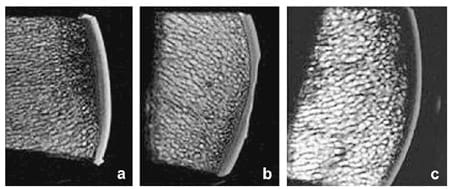
Fig. 3. Typical MRI images. (a) from the AC_AC_with meniscus group where there was no detectable change in wear volume at 78 µm x 78 µm in-plane pixel resolution (b) the AC_AC_meniscectomy group where surface damage was detectable at 78 µm x 78 µm in-plane pixel resolution and (c) a high resolution scan 20 µm x 20 µm in-plane pixel resolution of an undamaged sample.
Our studies and others8,10,12-14 have shown the importance of the level of contact stress and the nature of the loading cycle particularly the loadingeunloading cycle and the ability of AC to rehydrate during unloading11. In the current study, the limited flexioneextension motion of 25°, resulting in a shorter stroke length, may have limited the rehydration of the cartilage tissue, in comparison to that which occurs in vivo, causing a rise in frictional coefficient. Previous studies have shown how a shorter stroke length results in decreased fluid load support and increased friction11,24. Following meniscectomy, the decreased congruency which leads to larger localised contact stress, results in a more rapid decrease of the biphasic fluid load support, and subsequently, an increase in the coefficient of friction, the friction shear stress and the degradation of AC. This study supports our hypothesis that removal of the meniscus substantially diminishes the biphasic lubrication mechanism leading to rapid surface damage wear and permanent deformation at physiological load levels.
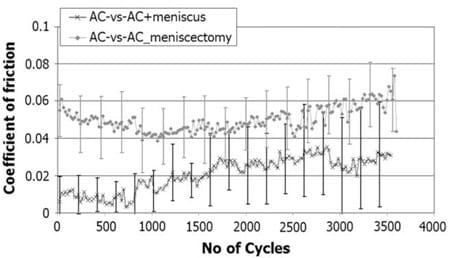
Fig. 4. Coefficient of friction over 3600 cycles for AC-vs-AC þ meniscus and AC-vs-AC_meniscectomy, at a peak load of 250 N. Data presented as mean (n ¼ 6) = 95% CI. There was a statistical difference ( P < 0.02; ANOVA single factor + T-Method) between the mean coefficients of friction (averaged over the 3,600 cycles).
It must be acknowledged at this point, that the loading applied to the meniscectomised bearing may have been quite severe. Clinically, meniscectomy results in a shift in the loading distribution between the two condyles, increasing the load supported by the intact compartment25. However, in the current simulat
ion, this shift in load distribution was not accounted for and the same level of loading was applied to the intact and meniscectomised bearings, meaning the worst case scenario was tested.
A steady rise in friction was found over the duration of the tests. The maximum average contact stresses in this study ranged between 2.8 MPa and 17.1 MPa depending on which bearing was tested and the level of loading applied. In a previous pin-on-plate study, performed at lower contact stress, no such rise in friction was found at up to 8 h15. This indicated that higher contact stresses may have caused some increase in friction, with the breakdown of AC constituents such as proteoglycan or collagen structure and/or the depletion of fluid load support, as stated by the biphasic theory. This has been supported by other studies which have indicated that the frictional force and wear are dependent on the loading cycle and contact stress11,13. An increase in the coefficient of friction has been shown to result from the loss of fluid support and increased direct solid-to-solid contact, due to the unique biphasic nature of AC8,15. Consequently wear increased as a result of direct solid-to-solid contacts. Meniscectomy can cause cartilage damage and fibrillation in the short term as well as in long term osteoarthritis. A number of animal studies have reported the tibiofemoral joint to show degenerative changes such as permanent displacement of matrix material, cartilage softening and cartilage lesions shortly after a total meniscectomy26-28.
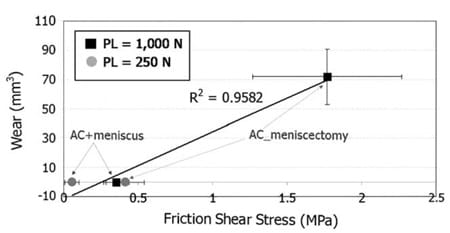
Fig. 5. Average friction shear stress vs cartilage wear volume, determined using mMRI scans. Data presented as mean (n = 6) ± 95% CI.
There were three forms of cartilage damage identified in this study: mild biomechanical wear in the form of surface fibrillation along with permanent displacement of matrix material, which were quantified using surface roughness measurements and the mMRI scans. It is presumable that there was also some degree of permanent deformation, associated with loss of matrix material and water. However, we have not to date been able to quantify the contributions to permanent deformation, but future studies will include analysis of matrix elements, such as collagen and proteins, in the lubricant.
The current simulation model has three limitations. Firstly, it only has unidirectional motion. Secondly, the swing phase motion of the gait cycle was reduced to 25°, consequently the available area for cartilage rehydration was limited, which may have caused an earlier breakdown in fluid load support, and hence a rise in frictional coefficient, which has been demonstrated in previous studies. This breakdown of fluid load support and elevation of friction coefficient has been demonstrated in previous studies11,24. Finally, the simulation only uses a 15 mm transverse section of the medial condyle. Future simulations will be advanced to include full gait, multiaxis kinematics and motions and whole natural joints using methods described for joint replacements. These experiments will allow the investigation of the effects of uni-compartmental meniscectomy, in terms of load and stress distribution and the wear of cartilage, both in the operated and non-operated compartmental can be examined. Bilateral meniscectomy will also be investigated. All of these future studies will include methodologies to characterise the detailed spatial and time-dependent pressure and shear stress distributions. Furthermore, computational studies, which are under development, will investigate localised stress and friction levels within the joint.
This study is the first tribological simulation of the medial compartmental knee and has many advantages over simple geometry configurations. More physiologically relevant contact stresses and motion can be applied, the geometry is that of the natural situation and the important biomechanical structure that is the meniscus can be included. A tribological simulation of this scale can be used to examine the effects of any potential intervention for osteoarthritis of the knee such as lubricants, meniscectomy repair or cartilage defect repair. The current work has shown for the first time, the direct elevation of the coefficient of friction, immediate surface fibrillation and biomechanical wear and permanent deformation of AC upon removal of the meniscus. This study further supports retaining the meniscus whenever possible in knee joint surgery and presents for the first time an in vitro model simulation system to investigate the tribological effects of meniscectomy and meniscus repair and regeneration.
Conflict of interest
Although DePuy International provided some of the funding for this project, all of the work was undertaken in the University of Leeds, and DePuy had no influence on the experimental work or analysis of results.
Professor John Fisher and Professor Eileen Ingham are the scientific directors of Tissue Regenix, a spin off company of the University of Leeds, which has an interest in acellular xenogeneic scaffolds. Tissue Regenix had no part in this study.
Acknowledgements
The authors would like to acknowledge EPSRC and DePuy International for funding this work. Thanks to our laboratory technicians, Phil Wood and Adrian Eagles.
References
- Fairbank TJ. Knee joint changes after meniscectomy. J Bone Joint Surg Br 1948;30-B:664e70.
- Klompmaker J, Jansen HW, Veth RP, Nielsen HK, DeGroot JH, Pennings AJ, et al. Meniscal repair by fibrocartilage? An experimental study in the dog. J Orthop Res 1992;10(3):359e70.
- McDermott ID, Sharifi F, Bull AMJ, Gupte CM, Thomas RW, Amis AA. The consequences of meniscectomy. J Bone Joint Surg Br 2006; 88-B:1549e56.
- Kettlekamp DB, Jacobs AW. Tibiofemoral contact areas e determination and implications. J Bone Joint Surg Am 1972;54:349e56.
- Seedhom BB, Dowson D, Wright V. Functions of the menisci e a preliminary study. J Bone Joint Surg Br 1974;56-B:381e7.
- Walker PS, Erkman MJ. The role of the menisci in force transmission across the knee. Clin Orthop Relat Res 1975;109:184e92.
- Krause WR, Pope MH, Johnson RJ. Mechanical changes in the knee after meniscectomy. J Bone Joint Surg 1976;58A(5):599e604.
- Forster H, Fisher J. The influence of loading time and lubricant on the friction of articular cartilage. Proc Inst Mech Eng 1996;210: 109e19.
- Forster H, Fisher J. The influence of continuous sliding and subsequent surface wear on the friction of articular cartilage. Proc Inst Mech Eng 1999;213:329e45.
- Krishnan R, Kopacz M, Ateshian GA. Experimental verification of the role of interstitial fluid pressurization in cartilage lubrication. J Orthop Res 2004;22:565e70.
- Bell CJ, Ingham E, Fisher J. Influence of hyaluronic acid on the timedependent friction response of articular cartilage under different conditions. Proc Inst Mech Eng [H] 2006;220:23e31.
- Carter MJ, Basalo IM, Ateshian GA. The temporal response of the friction coefficient of articular cartilage depends on the contact area. J Biomech 2007;40(14):3257e60.
- Katta J, Pawaskar SS, Jin ZM, Ingham E, Fisher J. Effect of load variation on the friction properties of articular cartilage. Proc Inst Mech Eng [J] 2007;221:175e81.
- Northwood E, Fisher J, Kowalski R. Investigation of the friction and surface degradation of innovative chondroplasty materials against articular cartilage. Proc Inst Mech Eng [H] 2007;221(3):263e79.
- Northwood E, Fisher J. A multi-directional in vitro investigation into friction, damage and wear of innovative chondroplasty materials against articular cartilage. Clin Biomech 2007;22(7):834e42.
- Mow VC, Hayes WC. Ba
sic Orthopaedic Biomechanics. 2nd edn. Lippincott-Raven; 1997. - McCutchen CW. Mechanism of animal joints: sponge-hydrostatic and weeping bearings. Nature 1959;184:1284e5.
- McCutchen CW. The frictional properties of animal joints. Wear 1962;5: 1e17.
- Mow VC, Kuei SC, Lai WM. Biphasic creep and stress relaxation of articular cartilage in compression: theory and experiments. J Biomech Eng 1980;102:73e84.
- Mow VC, Holmes MH, Lai WM. Fluid transport and mechanical properties of articular cartilage: a review. J Biomech 1984b;17(5): 377e94.
- McCann L, Udofia I, Graindorge S, Ingham E, Jin Z, Fisher J. Tribological testing of articular cartilage of the medial compartment of the knee using a friction simulator. Tribol Int 2008;41(11):1126e33.
- Simon WH. Scale Effects in Animal Joints. I. Articular Cartilage Thickness and Compressive Stress. Arthritis Rheum 1970;13(3): 244e55.
- DiStefano VJ. Function, post-traumatic sequelae and current concepts of management of knee meniscus injuries: a review article. Clin Orthop 1980;143.
- Caligaris M, Ateshian GA. Effects of sustained interstitial fluid pressurization under migrating contact area, and boundary lubrication by synovial fluid, on cartilage friction. Osteoarthritis Cartilage 2008;16(10):1220e7.
- Ihn JC, Kim SJ, Park IH. In-vitro study of contact area and pressure distribution in the human knee after partial and total meniscectomy. Int Orthop 1993;17(4):214e8.
- Bruns J, Kahrs J, Kampen J, Behrens P, Plitz W. Autologous perichondral tissue for meniscal replacement. J Bone Joint Surg Br 1998; 80B(5):918e23.
- Kohn D, Wirth CJ, Reiss G, Plitz W, Maschek H, Erhardt W, et al. Medial meniscus replacement by a tendon autograft. Experiments in sheep. J Bone Joint Surg Br 1992;74(6):910e7.
- Szomor ZL, Martin TE, Bonar F, Murrell GAC. The protective effects of meniscal transplantation on cartilage e an experimental study in sheep. J Bone Joint Surg Am 2000;82A(1):80e8.