Gordon R. Plank, Daniel M. Estok II, Orhun K. Muratoglu, Daniel O. O’Connor, Brian R. Burroughs, William H. Harris
Harris Orthopaedic Biomechanics and Biomaterials Laboratory, Massachusetts General Hospital, 55 Fruit Street, Jackson 1126, Boston, Massachusetts 02114
Abstract
Stress magnitude and distribution of both conventional polyethylene versus a crosslinked polyethylene in the liner of a total hip replacement (THR) were examined using finite element analysis and pressure sensitive film. Both types of polyethylene were assessed against head sizes of 22 and 28 mm with 5-mm thick polyethylene liners and head sizes of 28, 38, and 46 mm with 3-mm thick polyethylene liners. Liners with 5-mm conventional polyethylene represented successful combinations with long track records. Our hypothesis was that although the combination of the large head and the lower modulus of the highly crosslinked polyethylene would lead to lower stresses, the stresses would be excessive if the liner was extremely thin at 3 mm. Von Mises stresses at the articulating surface of the highly crosslinked liners were lower, when compared to conventional polyethylene, in every THR size examined. Specifically, however, the 38- and 46-mm inner diameter (ID) highly crosslinked polyethylene even at the extreme of only 3-mm thick had lower stresses than the 22-mm ID conventional liner of 5-mm thickness. These data indicate that the use of a large head against highly crosslinked material even at 3-mm thickness results in lower stresses than in an existing conventional 22-mm head and 5-mm thick combination. Obviously, other considerations will influence the minimum thickness to be recommended. © 2006 Wiley Periodicals, Inc. J Biomed Mater Res Part B: Appl Biomater 80B: 1–10, 2007
Keywords: THR; FEA; Fuji Film; acetabular; polyethylene; crosslinked
Introduction
Information from three types of sources, namely long duration in vivo human use (10- to 22-year follow-up data),1–4 contemporary in vitro hip simulator studies5–9 and contemporary RSA studies10,11 supports the use of highly crosslinked ultra high molecular weight polyethylene (UHMWPE) in total hip replacement (THR) surgery as an alternative bearing with markedly reduced wear. Several of the highly crosslinked polyethylenes also show sufficient wear resistance in hip simulator studies that the general concept that wear is increased with increasing head size may no longer be applicable in these new materials. In hip simulator tests, wear of some highly crosslinked polyethylene liners appears to be independent of head size.12 Three forms of more highly crosslinked polyethylene (Longevity® and Durasul ® from Zimmer, Warsaw, IN, and Marathon® from DePuy/Johnson & Johnson, Warsaw, IN) have been approved by the FDA for use with head diameters greater than 32 mm. On the other hand, the increased radiation to achieve the high level of crosslinking and the postradiation melting, which is used to reduce the concentration of free radicals, also reduce some of the mechanical properties of these materials, including the modulus, yield strength, ultimate strength, toughness, and resistance to fatigue crack propagation.
New data have shown the substantial advantages of the use of head sizes larger than 32 mm in diameter13,14 in terms of stability, range of motion, and absence of component-tocomponent impingement. However, the use of larger head sizes within a given outside diameter of the acetabular component would require the use of thinner polyethylene liners. While both the use of larger head diameters and the lower modulus of elasticity would predict that contact stresses under these circumstances might be reduced, the use of a thinner polyethylene liner would raise the risk of high stresses within the liner, as shown by Bartel et al.15 These considerations urge the determination of stress magnitude and distribution in polyethylene liners of metal-backed acetabular components, contrasting conventional UHMWPE versus highly crosslinked, melted UHMWPE in a variety of head sizes and particularly in thin polyethylene liners.
The hypothesis of this study was that 5-mm thickness would be the minimum thickness appropriate for use with the highly crosslinked polyethylene and that 3-mm thickness would be too thin in terms of stress magnitude and distribution. We also postulated high local stresses at the locking mechanism. The stresses that occur at the locking mechanism are of particular interest, since there have been cases of failure of the rim of retrieved highly crosslinked polyethylene liners with a 40-mm inner diameter (ID) when they were placed in a well-fixed, but highly abducted acetabular shell.16 Contact stresses were determined by finite element analysis (FEA) for both the nominal dimensions of the liners and heads and for limits of the tolerance stack for these same nominal dimensions.
Materials and Methods
In this study, contact stresses were determined using FEA and pressure sensitive film recordings for conventional and highly crosslinked polyethylene with head diameters of 22 and 28 mm in conjunction with liner thickness of 5 mm and with head diameters of 28, 38, and 46 mm in conjunction with liner thickness of 3 mm. FEA techniques were used to investigate the stress distributions and magnitudes of five sizes of UHMWPE acetabular liners under simulated one-legged stance during gait. Two of the liner sizes studied had a nominal thickness of 5 mm with inside liner/outside shell diameters of 22/39 and 28/45. The selection of 22/39 was chosen as the polyethylene is 5-mm thick and a 22-mm head results in high contact stress, and 28/45 was chosen as it also has 5-mm thick polyethylene and is a commonly used combination clinically. Implants with 28/45 dimensions have been used clinically for many years with success.17 They were chosen as examples for contact stresses widely occurring in successful THR. The other three liner sizes were 28/41, 38/51, and 46/59 with a nominal liner thickness of 3 mm, representing very thin liner designs. These dimensions were chosen to evaluate the 28-mm head size with very thin polyethylene of both conventional and highly crosslinked, melted polyethylene and then to see the extremes of combinations using thin polyethylene and very large head diameters.
The finite element meshes were derived from an earlie
r model18 using the mesh generator True Grid (XYZ Scientific Applications). The elements of the shell and liner were modeled as 8-node solid hexahedrons, while the femoral head was modeled as an analytical surface. A mesh density analysis was performed to ensure accurate results by increasing the element density of the polyethylene by approximate factors of two until convergence was observed. Increasing the number of elements in the polyethylene uniformly from 26,208 to 59,232 resulted in a decrease of ~5% in observed stresses and contact pressures with the 28-mm head. A decrease of ~10% for the same parameters was noted with the 46-mm head. Further increasing the number of elements in the polyethylene to 100,800 resulted in an additional decrease in observed stresses and contact pressures of less than 1% with the 28-mm head and less than 5% with the 46-mm head. Therefore, adequate convergence was assumed with the polyethylene modeled with 59,232 elements. In addition to the analytical surface for the head, each model consisted of 68,736 elements and 77,968 nodes with 59,232 elements in the polyethylene and 9504 elements in the rigid body shell. The contact interfaces between the head and liner, and liner and shell were modeled as sliding contacts with a coefficient of friction of 0.01.19 The femoral head was loaded with 3000 N (674 lbs) at an angle of 40° from the axis of symmetry, approximating the maximum joint reaction force and direction (Figure 1) of a patient weighing 225 pounds (102 kg) during gait.20 An exploded view of the model for the size 46/59 is shown in Figure 2.
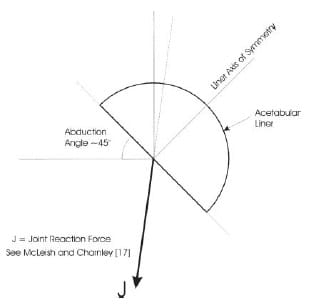
Figure 1. Joint reaction force diagram.
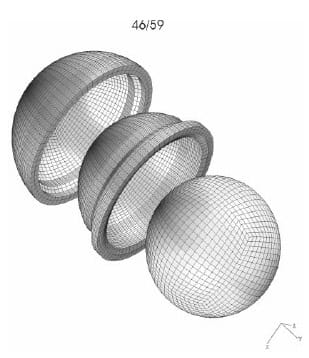
Figure 2. Exploded view of size 46/59 thin polyethylene THR assembly.
The two materials modeled were conventional UHMWPE, gamma sterilized (25–40 kGy) in nitrogen, and highly crosslinked UHMWPE, treated with e-beam irradiation (95 kGy) at a starting temperature of 125°C, followed by melting and sterilization with ethylene oxide (WIAM-95, i.e., warm irradiated with adiabatic melting and subsequent melt annealing). 5 Because the moduli of the shell and head are much higher than that of the polyethylene liner, these elements were modeled as rigid bodies. In so doing, there is virtually no loss in the accuracy of the stress and strain measurements within the polyethylene while avoiding the computational penalty that would occur (reduced time step–increased simulation time) if they were modeled as metals.
The liner inserts were modeled as elasto–plastic isotropic materials with properties based on measurements carried out under uniaxial compression. The properties used in the computation were piecewise linear approximations of the true stress and true strain illustrated in Figure 3. The elastic modulus and Poisson’s ratio in the linear range of the characteristics for the conventional UHMWPE were 873 MPa and 0.439 and for the highly crosslinked UHMWPE were 676 MPa and 0.425, respectively. The FEA software used was ABAQUS. The computational platform was a Dell Pentium IV (2.8 GHz) running Windows XP. Earlier work21 has suggested that nonconformity at the shell/liner interface may contribute more to backside wear of the liner than the presence of screw holes. For this reason, and to maintain simplicity, screw holes were not included in the model.
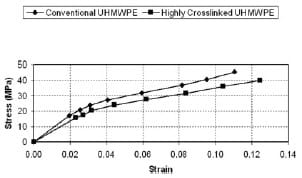
Figure 3. True stress/true strain conventional and highly crosslinked UHMWPE.
Each THR size was modeled two ways, (a) using nominal design dimensions, and (b) using one extreme of manufacturing tolerance stack values. The parameters adjusted to represent the extreme tolerance stack dimensions were the head, liner, and shell diameters at the head/liner and liner/ shell interfaces, while all other dimensions were held constant. Adjustment of the dimensions within manufacturing tolerances was done to create a situation that would result in elevated stresses at contact interfaces. Simplicity was maintained, however, by restricting these adjustments to the spherical surfaces of the THR. In reality, other dimensions may also vary, but the addition of those variations would result in a much larger and complex test matrix beyond the scope of the current exercise. To create the situation resulting from a tolerance stack extreme, the following reasoning was used. Reducing the diameter of the femoral head and increasing the inside diameter of the liner would be expected to increase the stress experienced at that interface. Similarly, decreasing the outside diameter of the liner and increasing the inside diameter of the shell would be expected to increase the stress experienced at that interface. The tolerance stack dimensions selected from the manufacturer’s data, therefore, consisted of minimum femoral head diameter, maximum liner inside diameter, minimum liner outside diameter, and maximum inside diameter of the shell. These conditions were applied simultaneously to each THR size modeled. The values for nominal and tolerance stack dimensions for each of the models are shown in Table I.
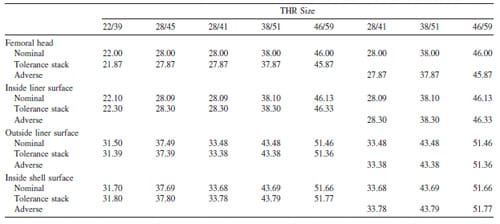
TABLE I. Values Used for Critical Diameters (mm)
The FEA test matrix consisted of two materials (conventional and WIAM-95 UHMWPE), two-dimensional configurations (nominal and tolerance stack), and four femoral head sizes (22, 28, 38, and 46 mm). As noted above, two head sizes (22 and 28 mm) were articulated against 5-mm polyethylene liners and three head sizes (28, 38, and 46 mm) were articulated against 3-mm thick liners for a total of 20 simulations. In the FEA model, the acetabular liner was loaded with 3000 N at an angle of 40° from the axis of symmetry representing single leg stance during gait. The load was ramped up to the maximum over 0.75 s and held there for 0.25 s.
Fuji Film contact pressure measurements were carried out with both conventional and highly crosslinked polyethylene and with the same liner/shell assembly sizes that were used in the finite element models. To create the thin (3-mm) polyethylene liners, production liners of larger sizes were reduced in thickness by the manufacturer to the requirements of the thin liners. Fuji Film Prescale measurements were made on three (3) samples of each combination of liner and head size used in the study. The acetabular liner was mounted in an MTS servo hydraulic testing machine and a load of 3000 N was applied in a direction 40° off the liner axis of symmetry (Figure 4) and held for 2 min. Pressure values reported here were the maximum encountered during loading. Under test conditions recommended by the manufacturer, the Fuji Film is accurate to within ±10%. The loading represented by both the FEA models and the Fuji Film tests was a single load applied to a fresh acetabular liner and thus did not consider creep or thermal effects.
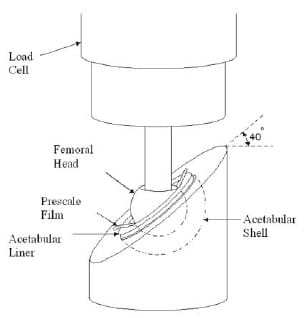
Figure 4. Test configuration for Fuji Prescale Film stress measurements.
Results
A summary of the results of the FEA stress analysis for the nominal and tolerance stack dimensional conditions is shown in Tables II and III,. The parameters reported are Von Mises stress, contact pressure, first principal stress, and shear stress in the plane of symmetry. Table IV lists the results of the contact pressure measurements with the Fuji Prescale Film on both the 5-mm polyethylene liners with 22- and 28-mm head sizes and the 3-mm polyethylene liners with 28-, 38-, and 46-mm head sizes. Typical contour plots of Von Mises stress at the head/liner articulating surface of the thin polyethylene liners for nominal and tolerance stack dimensions are shown in Figures 5 and 6, respectively. A typical plot of Von Mises stress at the liner/shell interface with tolerance stack dimensions is shown in Figure 7. A comparison of FEA results for nominal and tolerance stack interface dimensions is shown in Figure 8.
![Figure 5. Von Mises stress– head/liner articulating surface (nominal dimensions) 28/41–conventional polyethylene. [Color figure can be viewed in the online issue, which is available at www.interscience.wiley.com.] Figure 5. Von Mises stress– head/liner articulating surface (nominal dimensions) 28/41–conventional polyethylene. [Color figure can be viewed in the online issue, which is available at www.interscience.wiley.com.]](https://www.sensorprod.com/wp-content/uploads/2023/08/image05-5.jpg)
Figure 5. Von Mises stress– head/liner articulating surface (nominal dimensions)
28/41–conventional polyethylene. [Color figure can be viewed in the online issue,
which is available at www.interscience.wiley.com.]
![Figure 6. Von Mises stress– head/liner articulating surface (tolerance stack adverse dimensions) 28/41–conventional polyethylene. [Color figure can be viewed in the online issue, which is available at www.interscience.wiley.com.] Figure 6. Von Mises stress– head/liner articulating surface (tolerance stack adverse dimensions) 28/41–conventional polyethylene. [Color figure can be viewed in the online issue, which is available at www.interscience.wiley.com.]](https://www.sensorprod.com/wp-content/uploads/2023/08/image06-5.jpg)
Figure 6. Von Mises stress– head/liner articulating surface (tolerance stack
adverse dimensions) 28/41–conventional polyethylene. [Color figure can be viewed
in the online issue, which is available at www.interscience.wiley.com.]
![Figure 7. Von Mises stress–backside liner/shell interface (tolerance stack adverse dimensions) 28/ 41–conventional polyethylene. [Color figure can be viewed in the online issue, which is available at www.interscience.wiley.com.] Figure 7. Von Mises stress–backside liner/shell interface (tolerance stack adverse dimensions) 28/ 41–conventional polyethylene. [Color figure can be viewed in the online issue, which is available at www.interscience.wiley.com.]](https://www.sensorprod.com/wp-content/uploads/2023/08/image07-5.jpg)
Figure 7. Von Mises stress–backside liner/shell interface (tolerance stack
adverse dimensions) 28/ 41–conventional polyethylene. [Color figure can be viewed
in the online issue, which is available at www.interscience.wiley.com.]
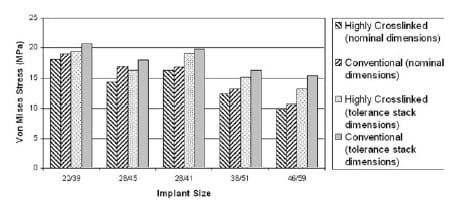
Figure 8. FEA results: nominal and tolerance stack adverse dimensions
contact pressure–head/liner interface (FEA and Fuji Film).

TABLE II. FEA Stress Analysis Data Summary (Nominal Dimensions)

TABLE III. FEA Stress Analysis Data Summary (Tolerance Stack Dimensions)

TABLE IV. Fuji Film Stress Analysis Data Summary
Overall, as delivered from the company, the dimensions of the manufactured liners were clustered around the tolerance stack limit dimensions discussed earlier. This fact is reflected in the values of maximum pressure gathered from the 3-mm polyethylene liners with the pressure sensitive film, that is, the maximum pressures measured are closer to the FEA values obtained for tolerance stack dimensions than for nominal dimensions (Figure 9). Figure 10 is a comparison of contact pressure film results for all of the liner sizes examined in this study.
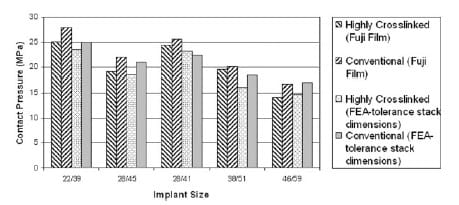
Figure 9. FEA (tolerance stack adverse dimensions)/Fuji Film comparison maximum
contact pressure (Fuji Film) highly crosslinked polyethylene.
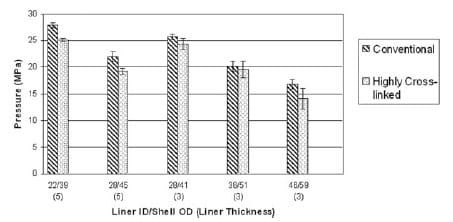
Figure 10. Fuji Film results summary maximum contact pressure.
Fuji Film tests with a commonly used THR assembly (28-mm head and 5-mm liner thickness) supported the FEA findings, demonstrating contact pressure levels of 19 MPa for highly crosslinked and 22 MPa for conventional material. This level for the conventional material is higher than all those measured with Fuji Film in the 3-mm polyethylene liners but with head diameters of 38 and 46 mm. Other Fuji Film pressure measurements were less than those of a 22-mm head against 5-mm thick conventional polyethylene.
Using the finite element models, under every test condition, the highly crosslinked UHMWPE experienced lower Von Mises stress levels than the conventional polyethylene at the contact interfaces and throughout the volume of the liner. The decrease in Von Mises stress levels was between 4 and 16%. With nominal dimensions, the stress distribution at the head/liner interface, as determined by FEA, was evenly distributed around a central location in the direction of the load (Figure 5). Under tolerance stack dimensional conditions, the stress levels were higher and the distribution was less uniform, with an additional area of elevated stress appearing closer to the rim of the liner as seen in Figure 6. The values presented in Tables II and III are for stresses and contact pressures computed at the head/liner articulating surface. Often, in the simulations of the tolerance stack conditions, high stresses and contact pressures were observed at locations on the equator, chamfer, or beneath the rim at the liner/shell interface (Figure 7).
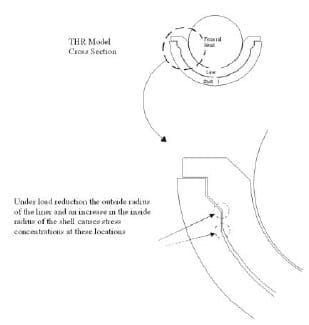
Figure 11. Location of stress concentrations under tolerance stack adverse case dimensions.
In the four way comparison of the FEA data for the head/liner articulating surface, using only 28-mm heads but comparing conventional and highly crosslinked material and comparing 3- and 5-liner thickness, the following was observed. In conventional polyethylene, all values (Von Mises stress, contact pressure, minimum principal stress, and shear stress) were higher in the 3-mm liners than in the 5-mm liners with one exception of Von Mises stress in the nominal dimension case. In the subset of highly crosslinked polyethylene, all values were higher in the 3-mm liners than in the 5-mm liners. Among only 5-mm liners, stresses were lower in the highly crosslinked polyethylene in every case. Among 3-mm liners, stresses were lower in the highly crosslinked polyethylene with the two exceptions of contact pressure and minimum principal stress in the tolerance stack dimension case.
In several instances, Von Mises stress in excess of the maximum occurring at the head/liner articulation occurred at various locations on the back side of the liner, usually on the equator or chamfer and occasionally under the rim in the general direction of the force application. In similar locations, the Von Mises stress on the back side of the liner was greater with conventional polyethylene than with highly crosslinked polyethylene. These areas of stress concentration occurred at regions of the locking mechanism of the shell (see Discussion).
While the stress contour patterns (Figures 5–7) were similar in both the conventional and highly crosslinked polyethylene, the Von Mises stress levels were consistently lower in the highly crosslinked material (Figure 8). Contact pressures measured with pressure sensitive film demonstrated higher pressures in the conventional polyethylene than in the highly crosslinked polyethylene in every case (Figure 10). In every case but one (Figure 9, 28/41, tolerance stack dimensions) contact pressures measured with FEA were also consistently higher in the conventional polyethylene than in the highly crosslinked polyethylene. Also, both the FEA and the pressure sensitive film methods, as anticipated, showed decreasing pressures with increasing head size for similar liner thickness for both the conventional and highly crosslinked polyethylene liners (Figures 9 and 10). The pressure sensitive film tests on the 22-mm head assembly with highly crosslinked polyethylene and a liner thickness of 5 mm showed a maximum contact pressure at the head/liner articulation of 25.1 MPa (Table IV). For this same 22-mm head assembly with conventional polyethylene, the maximum contact pressure measured was 27.9 MPa.
Discussion
The current study was performed to assess the stress levels and contact pressures experienced in THRs for 22- and 28-mm femoral heads under conditions of 5-mm thick liners and also for 28-, 38-, and 46-mm heads in the extreme case of thin (3-mm) polyethylene liners, employing nominal as well as tolerance stack dimensional conditions. In all but one test configuration modeled with FEA, the stresses and contact pressures computed for highly crosslinked polyethylene were consistently lower than those observed with conventional polyethylene. The maximum contact and Von Mises stresses computed for the 5-mm thick polyethylene liner were comparable to those computed previously by Bartel et al.15 and Kurtz et al.18 In the single case to the contrary (28/41, tolerance stack dimensions), the application of tolerance stack dimensions caused the assembly to exhibit high contact pressures under the rim of the liner, reducing the contact pressure somewhat at the head/liner articulating surface. Lower stresses and contact pressures in the highly crosslinked liners are consistent with the reduced modulus of this material. Examination of the stress contour plots consistently revealed slightly larger contact areas for the highly crosslinked UHMWPE compared to the conventional material. This is, of course, what physical principles would dictate, 15,22 given the equal force applied to both materials and the lower maximum stresses seen in the highly crosslinked UHMWPE.
In the FEA studies of tolerance stack dimensions, stresses were elevated in both the area of contact along the direction of the applied load and in an area nearer the rim of the liner (Figure 6). This stress elevation was apparent at both the femoral head/liner and liner/shell interfaces and appeared to originate at a corner where the contour of the metal shell made an abrupt transition from the ID of the shell to the rim, concentrating an increased proportion of the applied load at that location (Figure 7). Under tolerance stack dimensional conditions, this elevated stress at the backside of the liner often exceeded the stress seen at the head/liner interface. Figure 11 illustrates the THR crosssection and further details the location of the increase in the contact stress experienced at the backside of the liner under mismatched conditions. The local high stress levels seen in the tolerance stack dimensional conditions represent uniquely high values, which would likely soon be reduced by creep at those locations. One might speculate that with thicker liners, such stress transfer through the liner to the shell/liner interface would be less.
These data support that, from the point of view of contact stresses, when comparing equal thickness of the two materials, the highly crosslinked material has the advantage. But despite the decreased modulus of the highly crosslinked material, using a 3-mm liner with the same head size, resulted in higher stress levels. The highest stresses in both materials were often above the nominal yield stress limits of both highly crosslinked polyethylene and the conventional material (20 MPa, see Figure 3). The peak stress levels found in the study reflect in part, both the polyethylene thickness and the shell design. However, it is well known that other factors play a role in modifying the traditional relationships between excess stresses and deformation in the use of polyethylene in total joint applications, as Bartel et al. noted in their studies of conventional polyethylene.15 The creep that takes place early after implantation1,23,24 would likely reduce these stresses. The creep behavior of the two materials is nearly identical.23 In addition to the long term creep effect in reducing peak stresses for both the conventional and highly crosslinked polyethylene, the static FEA model does not take into account the thermal effects that may improve conformity at 37°C.
The asymmetrical distribution of stress (i.e., the occurrence of high stress levels near the rim away from the center of the force application) observed under tolerance stack dimensional conditions with FEA was not seen in the measurements with pressure sensitive film. This may point to limitations in the ability of the pressure sensitive film (given its thickness of 200 μm and potential edge effects) to measure such pressure gradients in a cavity of small diameter (22–46 mm) with minimal clearance between the femoral head and the liner. In addition, the slight differences noted in the measurements of stress with the Fuji Prescale Film versus the finite element analyses may be due to several factors such as (i) the material model used in the FEA is elasto–plastic with no viscoelasticity, or (ii) the Fuji Film is only accurate to ±10% under ideal conditions or both.
The expectat
ion that the highly crosslinked polyethylene would see lower stresses under parallel conditions is supported by the data from the comparisons of the 28-mm head series. However, our hypothesis that the stress levels in the very thin liners (3 mm) of the highly crosslinked polyethylene against head diameters 28–46 mm would be excessive was not supported.
The hypothesis that high stresses would occur at the locking mechanism was also supported. It has been shown that highly crosslinked polyethylene demonstrates a reduction in fracture toughness.25 Crosslinking at the radiation doses currently used for orthopedic devices increases the resistance to crack initiation, but decreases the resistance to crack growth or propagation.26 This is of significant interest in light of the findings at revision of a retrieved highly crosslinked polyethylene liner, which had a 40-mm ID and thus a relatively thin crosssection at the rim. The liner was implanted in a wellfixed socket, which was at an increased angle of abduction. At the time of revision, the rim was fractured at the location where the model predicts high levels of stress.16
These tests were done to assess contact stresses under clearly defined conditions of thin liners. The data shows that, contrary to our hypothesis, in the large head, thin (3-mm) polyethylene liner designs examined here, the changes in the mechanical properties of polyethylene induced by crosslinking and subsequent melting did not result in contact stresses greater than those found in current widely used implants with conventional polyethylene.
Other considerations, other than just stress states, such as other types of locking mechanisms, data on wear rates in vivo, data on wear rates in vivo with different head sizes, and data on different wear rates based on activity levels, will need to be superimposed on these data before a recommendation on minimum thickness can be made with safety.
References
- Wroblewski BM, Siney PD, Fleming PA. Low-friction arthroplasty of the hip using alumina ceramic and cross-linked polyethylene. J Bone Joint Surg Br 1999;81:54 –55.
- Oonishi H, Clarke IC, Masuda S, Amino H. Study of retrieved acetabular sockets made from high-dose, cross-linked polyethylene. J Arthroplasty 2001;16(8, Suppl 1):129 –133.
- Oonishi H, Kadoya Y, Masuda S. y-irradiated cross-linked polyethylene in total hip replacements-analysis of retrieved sockets after long-term implantation. J Biomed Mater Res 2001; 58:167–171.
- Grobbelaar CJ, Weber FA, Spirakis A, DuPlessis TA, Cappaert G, Cakic JN. Clinical experience with y irradiation-crosslinked polyethylene—A 14 to 20 year follow-up report. S Afr Bone Joint Surg 1999;XI(3):140 –147.
- Muratoglu OK, Bragdon CR, O’Connor DO, Jasty M, Harris WH. A novel method of cross-linking ultra-high-molecularweight polyethylene to improve wear, reduce oxidation and retain mechanical properties. Recipient of the 1999 HAP Paul Award. J Arthroplasty 2001;16:149 –160.
- Muratoglu OK, Bragdon CR, O’Connor DO, Jasty M, Harris WH. A comparison of five different types of highly cross-linked UHMWPEs: Physical properties and wear behavior. Trans Orthop Res Soc 1999;24:77.
- Muratoglu OK, Bragdon CR, O’Connor DO, Jasty M, Harris WH, Gul R, McGarry F. Unified wear model for highly crosslinked ultra-high molecular weight polyethylenes (UHMWPE). Biomaterials 1999;20:1463–1470.
- Muratoglu OK, Bragdon CR, O’Connor D, Perinchief RS, Estok DM II, Jasty M, Harris WH. Larger diameter femoral heads used in conjunction with a highly cross-linked ultra-high molecular weight polyethylene. J Arthroplasty 2001;16(8, Suppl 1):24 –30.
- McKellop HA, Shen F, Campbell P, Ota T. Effect of molecular weight, calcium stearate, and sterilization methods on the wear of ultra high molecular weight polyethylene acetabular cups in a hip joint simulator. J Orthop Res 1999;17:329 –339.
- Digas G, Karrholm J, Malchau H, Bragdon CR, Herberts P, Thanner J, Estok D, Plank G, Harris WH. RSA evaluation of wear of conventional versus highly cross-linked polyethylene acetabular components in vivo. Trans Orthop Res Soc 2003;28: 1430.
- Digas G, Karrholm J, Thanner J, Malchau H, Herberts P. Highly cross-linked polyethylene in cemented THA: Randomized study of 61 hips. Clin Orthop Relat Res 2003;417:126 –128.
- O’Connor DO, Bragdon CR, Muratoglu OK, Jasty M, Travers JT, Harris WH. The wear of one form of electron beam, highly crosslinked, melted UHMWPE is independent of head size in hip simulator testing. Trans Soc Biomater 2001;579.
- Burroughs BR, Golladay GJ, Hallstrom B, Harris WH. A novel constrained acetabular liner design with increased range of motion. J Arthroplasty 2001;16(8, Suppl 1):31–36.
- Burroughs BR, Rubash HE, Harris WH. Femoral head sizes larger than 32 mm against highly cross-linked polyethylene. Clin Orthop Relat Res 2002;405:150 –157.
- Bartel DL, Burstein AH, Toda MD, Edwards DL. The effect of conformity and plastic thickness on contact stresses in metalbacked plastic implants. J Biomech Eng 1985;107:193–199.
- Halley D, Glassman A, Crowninshield RD. Recurrent dislocation after revision total hip replacement with a large prosthetic femoral head. J Bone Joint Surg Am 2004:86:827– 830.
- Kay RM, Dorey FJ, Johnston-Jones K, Cracchiolo A, Amstutz HC, Finerman GA. Long-term durability of cemented primary total hip arthroplasty. J Arthroplasty 1995;10 (Suppl):S29 –S38.
- Kurtz SM, Giddings V, Muratoglu OK, O’Connor DO, Harris WH, Krevolin J. Stresses in a highly cross-linked acetabular component for total hip replacement. Trans Orthop Res Soc 2000;25:222.
- Simon SR, Radin EL. Lubrication and wear of the Charnley, Charnley-Muller, and McKee-Farrar prostheses with special regard to their clinical behaviour. In: Proceedings of the Hip Society, Saint Louis, MO; 1973.
- McLeish RD, Charnley J. Abduction forces in the one-legged stance. J Biomech 1970;3:191–209.
- Kurtz SM, Ochoa JA, Hovey CB, White CV. Simulation of initial frontside and backside wear rates in a modular acetabular component with multiple screw holes. J Biomech 1999;32:967–976.
- Bartel DL, Bicknell VL, Wright TM. The effect of conformity, thickness, and material on stresses in ultra-high molecular weight components for total joint replacement. J Bone Joint Surg Am 1986;68:1041–1051.
- Estok DM II, Bragdon CR, Plank GR, Huang A, Muratoglu OK, Harris WH. The measurement of creep in ultra-high molecular weight polyethylene. A comparison of conventional versus highly cross-linked polyethylene. J Arthroplasty 2005;20:239–243.
- Wroblewski BM, Siney PD, Dowson D, Collins SN. Prospective clinical and joint simulator studies of a new total hip arthroplasty using alumina ceramic heads and cross-linked polyethylene cups. J Bone Joint Surg Br 1996;78:280 –285.
- encur SJ, Rimnac CM, Kurtz SM. Failure micromechanisms during uniaxial tensile fracture of conventional and highly crosslinked ultra-high molecular weight polyethylene used in total joint replacements. Biomaterials 2003;24:3947–3954.
- Baker DA, Bellare A, Pruitt L. The effect of degree of crosslinking on the fatigue crack initiation and propagation resistance of orthopedic grade polyethylene. J Biomed Mater Res A 2003; 66:146 –154.