Craig M. Wiseman, MD,* Derek P. Lindsey, MS,† Amy D. Fredrick, MS,‡ and Scott A. Yerby, PhD*†‡
From the *San Francisco Orthopaedic Residency Program, San Francisco, California, †VA Rehab R&D Center, Palo Alto, California, and ‡St. Francis Medical Technologies, Alameda, California. Supported by the VA Rehab R&D Center, Palo Alto, CA, and St. Francis Medical Technologies, Alameda, CA. Acknowledgment date: January 5, 2004. First revision date: April 13, 2004. Second revision date: May 25, 2004. Acceptance date: May 28, 2004.
The device(s)/drug(s) that is/are the subject of this manuscript is/are being evaluated as part of an ongoing FDA-approved investigational protocol (IDE) or corresponding national protocol for neurogenic intermittent claudication secondary to lumbar spinal stenosis. Corporate/Industry funds were received in support of this work. One or more of the author(s) has/have received or will receive benefits for personal or professional use from a commercial party related directly or indirectly to the subject of this manuscript, e.g., honoraria, gifts, consultancies, royalties, stocks, stock options, or decision-making position.
Address correspondence and reprint requests to Scott Yerby, PhD, Director R&D, St. Francis Medical Technologies, Inc., 960 Atlantic Avenue, Suite 102, Alameda, CA 94501; E-mail: [email protected]
Study Design. Facet loading parameters of lumbar cadaver spines were measured during extension before and after placement of an interspinous process implant.
Objective. The study was undertaken to quantify the influence of an interspinous implant on facet loading at the implanted and adjacent levels during extension.
Summary of Background Data. Facet loading is increased during extension and decreased during flexion. Previous studies have demonstrated that interspinous process decompression relieves disc pressure at the implanted level and does not alter disc pressure at the adjacent levels. Facet joints are believed to play a key role in back pain, especially in patients with collapsed discs and increased motion segment mobility resulting in increased facet loading.
Methods. Seven cadaver spines (L2–L5) were loaded to 15 Nm of extension and 700 N compression with and without an interspinous process implant (X STOP) placed between the L3–L4 spinous processes. Pressure-sensitive film was placed in the facet joints of the implanted and adjacent levels. After loading, the film was digitally analyzed for peak pressure, average pressure, contact area, and force. These values were compared between the intact and implanted specimens at the adjacent and implanted levels using a paired t test (P < 0.05).
Results. The implant significantly reduced the mean peak pressure, average pressure, contact area, and force at the implanted level. The mean peak pressure, average pressure, contact area, and force at the adjacent levels were not significantly different between the intact and implanted specimens with the exception of contact area at the L2–L3 level.
Conclusions. Interspinous process decompression will unlikely cause adjacent level facet pain or accelerated facet joint degeneration. Furthermore, pain induced from pressure originating in the facets and/or posterior anulus of the lumbar spine may be relieved by interspinous process decompression. Clinical results from patients with a component of lower back pain suggest that this is a valid conclusion.
Key words: facet joint, neurogenic intermittent claudication, lumbar, back pain, interspinous process decompression. Spine 2005;30:903–907
Although the precise etiology of lower back pain (LBP) lacks a general consensus, there is general agreement that the facet joints must be considered when studying its pathology.1–7 The lifetime incidence of LBP is estimated to be between 60% and 90% with 15% to 40% of chronic LBP caused by the lumbar facet joints.8–10 Although clinically difficult to diagnose, facet specific back pain is exacerbated by hyperextension and lessened in a recumbent position or flexion.1,6,8 During extension, loading of the facet joints is increased, resulting in compression of the nerve root and central canals and deformation of the joint capsule.6,9 It is believed that the deformation of the joint capsule is the source of pain as it stimulates nociceptors of the capsule.8,11 The capsule surrounding the facet joint has been shown to be well innervated by afferent nociceptive fibers, which are activated by mechanical stresses.1,2,4,6,7,11
Several in vitro studies and analytical models have confirmed that facet loading is increased during extension. 5–8,12–15 Shirazi-Adl and Drouin showed that the facets carry as much as 30% of the load in the presence of 2° to 5.6° of extension rotation.16 Yang and King reported an increase in facet loading with an increasing extension moment; the facet loading could increase as high as 47% in an arthritic patient.1 Adams and Hutton have shown that slight flexion relieves the facet joint and the compressive force on the posterior anulus.17
The load-bearing structures in each vertebra of the spinal column form a tripod of support. The structures that make up each leg of this tripod consist of the vertebral body and each of the 2 facets. Loading between adjacent vertebral bodies is transmitted via the intervertebral discs, and the loading between adjacent pedicles is transmitted via the apophyseal, or facet, joints. All 3 legs of this tripod, with the intervertebral disc, ligaments, and facet joints make up the lumbar motion segment and act in concert to provide stability to the spinal column. 12,13,15,17–19 Structurally, this motion segment is important because it maintains alignment when exposed to flexion–extension, lateral bending, and/or rotational forces. With injury to 1 of these segments, the mechanical loads of the other structures are altered. Increased facet loading has been shown to be a consequence of disc degeneration, which in turn can lead to lumbar spinal stenosis.10,13,17,18 In the case of facet joint degeneration or removal, the segment is unstable in both axial rotation and flexion, allowing greater sagittal displacements, and has been shown to accelerate disc degeneration.14 Furthermore, it has been hypothesized that long-term lumbar segment instability results in degenerative spondylolisthesis. 8–10,16
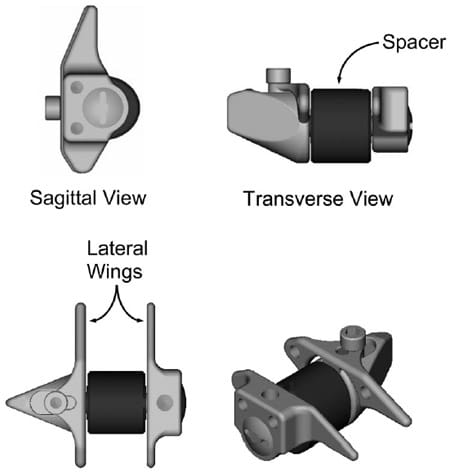
Figure 1. A schematic of the X STOP interspinous implant.</strong >
A novel interspinous implant (X STOP® Interspinous Process Decompression System, St. Francis Medical Technologies Inc., Almeda, CA) has been developed to treat lumbar neurogenic intermittent claudication (NIC) secondary to spinal stenosis. The implant prevents narrowing of the spinal canal and neural foramina during extension and is placed between the spinous processes from a lateral approach through the interspinous ligament. Its position is maintained by the supraspinous ligament posteriorly, the lamina anteriorly, and 2 wings located on the implant laterally (Figure 1). A possible concern of this implant is how it affects facet loading at adjacent levels. Increased facet loading may cause degenerative changes in the facet joints, which may in turn cause lower back pain.
The purpose of the current study is to quantify the effects of the X STOP implant on the loading of the facet joints during extension. It is hypothesized that the facet loading at the implanted level is significantly reduced without significantly affecting the loading at the adjacent levels.
Materials and Methods
Seven human lumbar (L2–L5) cadaver specimens were cleaned of all muscle and adipose tissue, and the ligamentous structures were left intact. The facet capsules of the left and right L2–L3, L3–L4, and L4–L5 facets were removed. The cranial portion of L2 and caudal portion of L5 vertebrae were secured in polymethyl methacrylate (PMMA), and each specimen was placed in a spinal loading frame capable of applying independent bending moments and axial loads (MTS Multi-Channel Spine Test Fixture, MTS Systems, Eden Prairie, MN). The number of specimens used in the current study was based on a power analysis of the results of el-Bohy et al.7
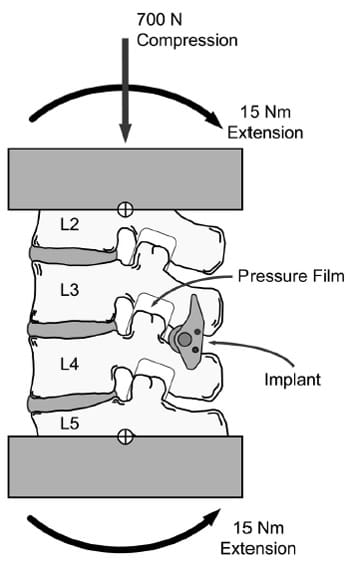
Figure 2. A schematic of the testing configuration. A 15 Nm bending moment was applied to each specimen via servohydraulic rotational actuators secured to the cranial and caudal ends of the specimen, and a 700 N axial load as applied via a servohydraulic linear actuator at the cranial end. The cross-hairs represent the centers of rotation for the cranial and caudal rotational actuators.
Before testing, all specimens were preloaded with 700 N of axial compression until the axial displacement stabilized. This generally occurred in between 15 and 60 minutes. Direct measurements of facet joint pressures and area were made by first placing ultra low range pressure-sensitive film (PressureX, Sensor Products, East Hanover, NJ) in the left and right facet joints at each level. The specimens were tested intact initially by first applying a 700 N compressive axial load at a rate of 25 N/sec followed by a 15 Nm extension moment applied at a rate of 0.25 Nm/sec (Figure 2). The peak load was held for 60 seconds. The loading regimen was based on published values16,18 as well as a series of pretrial experiments conducted to ensure consistent and repeatable facet loading measurements with the pressure- sensitive film. The 15 Nm extension bending moment was applied via servohydraulic rotational actuators placed at the cranial and caudal ends of the specimens and the 700 N axial load was applied via a servohydraulic linear actuator placed at the cranial end of the specimens. Following testing, if the film loading patterns (Figure 3) were found to exceed the film’s sensitivity range, the film was replaced with the next higher sensitivity range (super low, low, and medium), and the loading regimen was repeated until the film was no longer saturated. In general, area measurements were taken from the ultralow film and peak pressure measurements were taken from the highestgrade film. All specimens were tested using from 2 to 4 film grades.
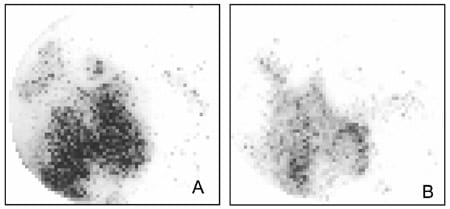
Figure 3. Representative loading patterns of a facet of (A) an intact motion segment and (B) the same motion segment instrumented with the X STOP.
Following the intact testing, the specimens were removed from the loading frame and an appropriately sizedXSTOP was implanted in the anterior portion of the interspinous process space at L3–L4 in each specimen. The specimens were fitted with new pressure-sensitive film, returned to the loading frame, and the previously described loading regimen was reapplied to each specimen.
Independent film calibration curves were created in an axial load frame using known applied loads and areas for each film grade used. After loading, the calibration films were scanned on a flat-bed scanner and converted to 8-bit gray scale images that were used to develop gray scale versus pressure calibration data for each film grade using image analysis software (Scion Image, Scion Corp., Frederick, MD). The gray scale versus pressure data were fit with a fourth order regression curve that was used to convert the test specimen film loading patterns to pressure and area measurements. Peak facet pressure was calculated as the greatest pressure from the highest film grade used for each specimen. The average facet pressure was calculated as a weighted average of the unsaturated regions of all films as described by Huang et al.20 This technique, in effect, superimposes all unsaturated pressures from all films to form a single pressure distribution that incorporates pressures collected from the lowest to the highest film grade used. The average pressure is then calculated as the weighted average of all unsaturated pressures. Contact area was calculated as the greatest contact area among all film grades used for each specimen. The mean force was calculated by multiplying the contact area and mean pressure. The mean values of peak pressure, average pressure, contact area, and force of the intact and implanted specimens were compared at the implanted and adjacent levels using paired t tests (P < 0.05).
Results
The X STOP significantly (P < 0.05) reduced the mean peak pressure, average pressure, contact area, and force at the implanted level (Table 1, Figure 4). The peak pressure was reduced 55% from 3.73 MPa to 1.68 MPa (P < 0.0021, power = 0.953). The mean pressure was reduced 39% from 0.93 MPa to 0.57 MPa at the implanted level (P<0.0002, power=0.999). The contact area was reduced by 46% from 0.79 cm2 to 0.42 cm2 at the implanted level (P < 0.0002, power = 0.999). The mean force was reduced by 67% from 83.2 N to 26.8 N (P < 0.0039, power = 0.912). The mean peak pressure, average pressure, contact area, and force at the adjacent levels were not significantly different between the intact and implanted specimens with the exception of contact area at the L2–L3 level (Table 1, Figure 4).
Discussion
The results of the current study demonstrate that the X STOP does not significantly affect the facet loading parameters at the adjacent levels and significantly decreases the facet loading parameters at the implanted level. These results suggest that the X STOP will unlikely cause accelerated facet degeneration due to increased facet loading at the adjacent levels. These results at the implanted level are consistent with those of Minns and Walsh, who predicted that insertion of an interspinous process implant would decrease facet loading at the implanted level.21 However, facet loading of the adjacent segments was not addressed, and direct measurements of the facet loading was not made. In fact, few studies have made direct measurement of the facet joints using pressure- sensitive film. Lorenz et al used pressure-sensitive film to quantify facet loading in normal and surgically altered segments.19 However, a different loading protocol was used, and only single-level motion segme
nts were tested, thus not allowing for a direct comparison of results to be made with the current study.19 Despite these differences, the contact area and facet load (force) measured in both studies are similar, and the peak pressure was approximated half of that measured in the current study. Also, when using pressure-sensitive film, a ± 15% error is associated with the pressure measurement; therefore, direct comparison to previous biomechanical studies that did not use pressure-sensitive film is somewhat difficult.22

Table 1. Average Peak Pressure, Mean Pressure, Contact Area, and Force
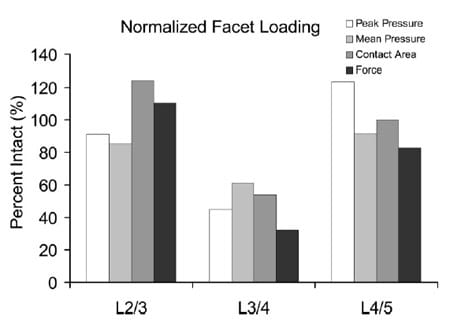
Figure 4. A bar chart of the mean peak pressure, average pressure, contact area, and force of the implanted specimen normalized to the intact specimen.
A limitation of this study is that only facet loading during extension was quantified, and loading in the neutral or flexed positions was not addressed. However, this limitation is not likely to have a significant consequence, as the highest compressive loads experienced by the facet joints occur during extension. Several studies have reported that the greatest loads experienced by the facet joints occur during extension.1–4,10,12,13,17
Current treatment options for facet-induced lower back pain include arthrodesis and therapeutic injection. Both treatment options have had limited success with long-term LBP relief. Arthrodesis has been reported as an unreliable method to treat facet-induced back pain, and outcomes measures have shown little association between arthrodesis and facet-induced pain relief due to the difficulty in accurately identifying the origin of the pain.9,23 Berven et al have reported that in 2 randomized controlled studies, intra-articular steroid injections have had no benefit in treating facet-inducted lower back pain.9 In noncontrolled clinical studies, the effect of intra- articular steroid injections has been variable. Success rates for long-term pain relief (greater than 6 months) have been reported to be between 18% and 63% in the noncontrolled studies.8,9
The aim of the current study was to quantify the effect of interspinous decompression on facet loading. This study has demonstrated that the X STOP significantly reduced facet loading at the implanted level and did not alter facet loading at the adjacent levels. These results are consistent with those of Swanson et al, who showed that interspinous process decompression significantly reduced disc pressure at the implanted level, but did not significantly affect disc pressures at the adjacent levels.24 Furthermore, Lindsey et al showed that flexion and extension range of motion were significantly reduced, but axial rotation and lateral bending were unaffected at the implanted level.25 At the adjacent levels, flexionextension, axial rotation, and lateral bending were not significantly affected. This loading reduction reported in the current study combined with the pressure reduction of the posterior disc, and no change in axial rotation or lateral bending range of motion suggests that interspinous process decompression may be effective in treating load-induced back pain originating in the posterior anulus and/or facets.
Key Points
- A biomechanical study quantified the influence of an interspinous process implant on facet loading at the implanted and adjacent levels during extension.
- Interspinous process decompression significantly reduced facet loading at the implanted level, but did not significantly alter the loading at the adjacent levels with the exception of contact area at L2–L3.
- Interspinous process decompression may reduce pressure-induced pain in the facet and/or posterior anulus of the lumbar spine.
References
- Yang KH, King AI. Mechanism of facet load transmission as a hypothesis for low-back pain. Spine 1984;9:557–65.
- Yamashita T, Minaki Y, Ozaktay AC, et al. A morphological study of the fibrous capsule of the human lumbar facet joint. Spine 1996;21:538–43.
- Yamashita T, Cavanaugh JM, Ozaktay AC, et al. Effect of substance P on mechanosensitive units of tissues around and in the lumbar facet joint. J Orthop Res 1993;11:205–14.
- Wyke BD. Structural and functional characteristics of articular receptor systems (author’s translation). Acta Chir Orthop Traumatol Cech 1973;40: 489–97.
- Avramov AI, Cavanaugh JM, Ozaktay CA, et al. The effects of controlled mechanical loading on group-II, III, and IV afferent units from the lumbar facet joint and surrounding tissue. An in vitro study. J Bone Joint Surg Am 1992;74:1464–71.
- Cavanaugh JM, Ozaktay AC, Yamashita HT, et al. Lumbar facet pain: biomechanics, neuroanatomy and neurophysiology. J Biomech 1996;29: 1117–29.
- el-Bohy AA, Yang KH, King AI. Experimental verification of facet load transmission by direct measurement of facet lamina contact pressure. J Biomech 1989;22:931–41.
- Dreyer SJ, Dreyfuss PH. Low back pain and the zygapophysial (facet) joints. Arch Phys Med Rehabil 1996;77:290–300.
- Berven S, Tay BB, Colman W, et al. The lumbar zygapophyseal (facet) joints: a role in the pathogenesis of spinal pain syndromes and degenerative spondylolisthesis. Semin Neurol 2002;22:187–96.
- Sharma M, Langrana NA, Rodriguez J. Role of ligaments and facets in lumbar spinal stability. Spine 1995;20:887–900.
- Yamashita T, Cavanaugh JM, el-Bohy AA, et al. Mechanosensitive afferent units in the lumbar facet joint. J Bone Joint Surg Am 1990;72:865–70.
- Abumi K, Panjabi MM, Kramer KM, et al. Biomechanical evaluation of lumbar spinal stability after graded facetectomies. Spine 1990;15:1142–7.
- Adams MA, Hutton WC. The effect of posture on the role of the apophysial joints in resisting intervertebral compressive forces. J Bone Joint Surg Br 1980;62:358–62.
- Haher TR, O’Brien M, Dryer JW, et al. The role of the lumbar facet joints in spinal stability. Identification of alternative paths of loading. Spine 1994;19: 2667–70, discussion 2671.
- Kato Y, Panjabi MM, Nibu K. Biomechanical study of lumbar spinal stability after osteoplastic laminectomy. J Spinal Disord 1998;11:146–50.
- Shirazi-Adl A, Drouin G. Load-bearing role of facets in a lumbar segment under sagittal plane loadings. J Biomech 1987;20:601–13.
- Adams MA, Hutton WC. The relevance of torsion to the mechanical derangement of the lumbar spine. Spine 1981;6:241–8.
- Dooris AP, Goel VK, Grosland NM, et al. Load-sharing between anterior and posterior elements in a lumbar motion segment implanted with an artificial disc. Spine 2001;26:E122–9.
- Lorenz M, Patwardhan A, Vanderby R, Jr. Load-bearing characteristics of lumbar facets in normal and surgically altered spinal segments. Spine 1983; 8:122–30.
- Huang A, Hull ML, Howell SM. The level of compressive load affects conclusions from statistical analyses to determine whether a lateral meniscal autograft restores tibial contact pressure to normal: a study in human cadaveric knees. J Orthop Res 2003;21:459–64.
- Minns RJ, Walsh WK. Preliminary design and experimental studies of a novel soft implant for correcting sagittal plane instability in the lumbar spine [see comments]. Spine 1997;22:1819–25; discussion 1826–7.
- Sensor Products Inc. Color Correlation Manual for Pressure Int
erpretation. East Hanover, NJ: Sensor Products; 2003. - Axelsson P, Johnsson R, Stromqvist B, et al. Posterolateral lumbar fusion. Outcome of 71 consecutive operations after 4 (2–7) years. Acta Orthop Scand 1994;65:309–14.
- Swanson KE, Lindsey DP, Hsu KY, et al. The effects of an interspinous implant on intervertebral disc pressures. Spine 2003;28:26–32.
- Lindsey DP, Swanson KE, Fuchs P, et al. The effects of an interspinous implant on the kinematics of the instrumented and adjacent levels in the lumbar spine. Spine 2003;28:2192–7.