Kalpana R. Agrawal* and Peter W. Lucas
An analysis of the action of the incisor teeth in humans is presented in terms of the fracture of food particles. It is predicted that the resistance of foods with an essentially linear elastic response to an initial bite by the incisors will depend on the square root of the product of two food properties, Young’s modulus and toughness. This quantity should be approximately equal to the product of the stress at cracking during a bite, and the square root of the length of a notch or indentation from which that crack initiates. As a test of the theory, the relationship between in vivo stresses and the depth of incisal penetration, measured during bites on seven ‘snack’ foods by 10 subjects, and food properties established from mechanical testing, was investigated. Theory and experiment were found to be in excellent agreement. A dimensionless index of the efficiency of incision is suggested, relating fracture performance by subjects to values from a testing machine. This appears to have a high level of inter-subject discrimination with efficiencies varying about threefold. The method appears to have potential applications in dentistry, food science and studies of human and primate evolution.
Keywords: incisors; foods; fracture; bite mechanics
1. INTRODUCTION
Among living mammals, spatulate incisor teeth in both upper and lower jaws are unique to the Anthropoidea, a taxonomic group containing the apes, Old World and New World monkeys. These teeth are relatively large in fruit-eating primate species (Hylander 1975), making it probable that the trait evolved in association with this type of diet. However, humans use their incisor teeth on a much more diverse range of foods than fruits in order to control the size and shape of ingested food particles. The teeth are of specific interest to researchers in two major disciplines. A clinical speciality in dentistry called orthodontics deals with the adjustment of tooth orientation. Although the orthodontic treatment of incisors is usually indicated by aesthetic considerations, functional analysis suggests that a vertical incisal inclination, generally the desired clinical outcome, may be out of alignment with the general direction of muscular force (Paphangkorakit & Osborn 1997). There have also been a large number of studies by food scientists of incision related to consumer preference. The acceptability of many classes of food to consumers is strongly influenced by expectations about food texture. Texture can be evaluated manually, but perceptions concerning fracture involve the teeth and the first bite with the incisors seems critical in formulating opinions (Bourne 2002). Exactly how such sensations are perceived is unknown and analyses have centred on the correlation of psychophysical responses with the mechanical characteristics of foods, the latter as obtained from often rather arbitrary tests on universal testing machines. Significant correlations between a perceived food quality and a mechanical property should not imply a functional relationship because if only a limited range of foods is offered, their mechanical properties would be likely to be correlated with each other (Ashby 1998). Spurious correlations may follow, which may well break down over a broader range of foods.
Most uses of the teeth involve the fracture of foods. However, food scientists have mainly ignored the theory of fracture in their mechanical characterizations. Applying fracture mechanics, Vincent et al. (2002) found that the critical stress intensity factor, KIC, of foods, a parameter related to crack initiation, was linearly related to perceived measures of ‘hardness’ and ‘crunchiness’ as evaluated by trained ‘taste’ panellists. This paper explores this fracture criterion further by comparing it with in vivo conditions, the aim being to provide a theoretical basis for incision and an index of dental efficiency that could be used for studies of incisor function in humans.
2. THEORETICAL BACKGROUND
Usually, in an incisal bite, a hand holds a fruit like an apple close to the edges of the upper incisors while the lowers are swung upwards towards it causing, first, an indentation on the lower surface (figure 1a), followed by cracking (figure 1b). The upper incisors may also move due to the action of the neck muscles, which can influence the food surface on which a crack is initiated (Osborn et al. 1987). Our analysis is close to that of Osborn et al. except that it is not based on fracture stress, but on energy. Any fracture event can be analysed in two ways: (i) in terms of the balance between the strain energy within the particle and the energy required to extend the crack from the indentation; or (ii) in terms of the effect on the stresses in the apple caused by the presence of the indenting incisors. We can model this generally only for a quasi-static elastic fracture, in which the fractured surfaces still fit together to resemble the original and where the crack growth is relatively slow. The propagation of a crack initiated from the indentation formed by the lower incisors will be at a critical value of the Irwin stress intensity, KIC, given by where the fracture stress is sF , C1 is a constant and a is the depth of indentation of the lower incisor (Atkins & Mai 1985). Provided the stress–strain curves of foods are essentially linear, then another way of expressing the same result is the use of the Griffith equation for the energy balance within the apple tissue

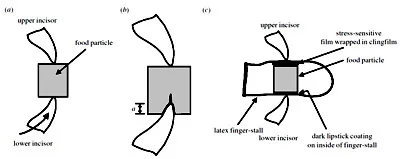
Figure 1. (a) A food particle (grey) is held against the upper incisors. Movement of the lower incisors relative to the food produces an indentation from which, in (b), a crack eventually initiates. In (c), the experimental set-up is shown. Stresses are registered on the upper face of the food particle, remote from the crack, by a two-layered pressure-sensitive film. The film is covered in plastic wrap and both food particle and wrapped film are then enclosed in a 0.4 mm thick latex finger-stall. Posttest measurement of the penetration of the lower incisor was aided by painting either the lower surface of the food particle or the inside of the finger-stall with lipstick.

where E is the Young’s modulus and R is the toughness of the apple tissue (Atkins & Mai 1985). C2 is another dimensionless constant where, in idealized loading conditions in which the load is applied remote to a crack in a large body, C1 = C2 = π0 .5 . These conditions hardly apply to the action of the teeth and, therefore, in combi
ning equations (2.1) and (2.2), we must define a new constant depending on the loading geometry:

If, on the one hand, we can measure the stresses in the food particle at cracking in vivo and measure the food properties, Young’s modulus and toughness, with mechanical testers, then we can use a fourth relationship

to test whether KIC forms an effective criterion for crack growth during an incisal bite. The material properties on the right-hand side of equation (2.4) can be measured easily on a universal testing machine. The issue is whether the factors on the left-hand side, the in vivo stress at cracking and the depth of indentation, can also be measured. If so, then a straight-line relationship is predicted, passing through the origin, with the dimensionless constant, C4 , being given by the slope of a graph where σFa0 .5 is plotted against (ER)0 .5 . This constant offers a measure of incisal efficiency, defined as the ratio of in vivo fracture to ‘in vitro’ values obtained from machine tests. The lower the value of C4 , the greater is the efficiency of the lower incisors. The efficiency of the upper incisors could be tested separately if conditions can be organized such that they alone penetrate the food particle to cause cracking directed from its upper surface.
3. MATERIAL AND METHODS
Foods were four types of cheese, raw carrot, Brazil nut and macadamia nut kernels (in table 1). The toughness, R, of the cheeses and carrot were obtained with a 15° included angle sharp stainless steel wedge driven into a rectangular block of food (Khan & Vincent 1993) at a crosshead speed of 140 mm min21. The test is shown schematically in figure 2a. From point A, the force built with indentation until cracking at B. The crack was unstable at first but quickly settled (point C). Further movement of the wedge between C and D produced a stable crack running just ahead of the wedge tip. The area under the curve was measured between C and D. The wedge was then reversed to position A and run through the cracked specimen producing a friction curve labelled AEF. The mean friction varied between 5.8% (carrot) and 25.0% (reduced fat cheddar) of the total work done. The area bounded by CDFE gave the work done to produce the crack. Nut kernels were too brittle for crack growth to be controlled in this test. Therefore, the nuts were sectioned to a thickness of 0.15–0.88 mm and then cut using scissors (Dovo, Germany) using a portable tester (Darvell et al. 1996) equipped with a motor producing cutting speeds of 30–50 mm min2 1. The tests, shown in figure 2b, showed no apparent dependence of toughness on section thickness, but both nuts were tested over a similar thickness range as a precaution. Only tests in which the crack was controlled to the end of the specimen were classed as valid. The Young’s modulus, E, of the foods was obtained by compressing cylindrical or cuboid specimens (made with cork borers or dies) of low aspect ratio on a Lloyd LRX (UK) universal testing machine at a crosshead speed of 5 mm min2 1. A thin adhesive-backed Teflon strip attached to the compression plates served to reduce friction.
For the bite tests, foods were shaped into rectangular blocks, measuring 12 mm (height) ´ 12 mm ´ 20 mm for cheeses and carrot or 8 mm (height) ´ 10 mm ´ 14 mm for the nuts. The 12 mm width of the block (figure 3a) was considerably smaller than the sum of the mesiodistal length of the four lower incisors, averaging ca. 20 mm.
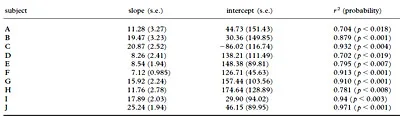
Table 1. Statistics of the in vivo data regressed against food properties from machine tests for individual subjects.
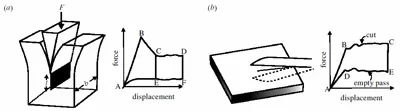
Figure 2. (a) Diagram of the configuration of a wedge test, designed to estimate the toughness of specimens of cheeses and carrot. The test involved two passes of the wedge, one to initiate and grow a crack just ahead of the wedge tip (marked by ABCD) and the second, to deduct the work of friction by passing the wedge against the fractured surface (AEF). The length of the crack could be estimated from the displacement of the wedge. The area CDFE on the force–displacement graph encloses the work related to the fracture and corresponds to the fractured area (bt) shown in black at left. (b) Diagram to show an analogous test with scissors on thin sections of nut kernels. The graph area ABCED gives the work done. Specimens were cut through completely to simplify measurement of the crack area. For both tests, the work done divided by the crack area gave toughness.
Ten subjects (five males, five females, aged 22–49 years, mean of 31 years, s.d. of 8 years) made one bite on a standard sized food particle enclosed in a 0.4 mm thick latex finger-stall (a latex finger-tip protector). The finger-stall shielded the food’s identity from subjects and prevented salivary contamination (Agrawal et al. 1997; Lucas et al. 2002). Subjects were asked to pick the particle up by hand, place this between the incisors and bite normally, but to stop just as a particle ‘gave way’, i.e. cracked (figure 1c). This last part of the instruction was required to avoid the recording of stresses at tooth–tooth contact, which could easily have exceeded the stresses during food loading. No subject had difficulty with this instruction and the teeth did not carry through to tooth–tooth contact. The cracks in three of the cheeses and raw carrot usually arrested within the food block, but those in Parmesan cheese and nut kernels continued in order to subdivide the particles.
Kohyama & Nishi (1997) pioneered in vivo measurement of the pressure distribution on food surfaces during incision. The sheet sensors they used are very thin and flexible (less than 0.1 mm; Kohyama et al. 1997), but produce a continuous electrical signal that can be converted to pressure via calibration curves, provided the sheet remains flat during a bite. Instead, being interested only in the conditions at fracture, we employed a pressure-sensitive film (Fuji Prescale, Japan) that is thicker and stiffer than a sheet sensor, but can only record the maximum compressive stress during the test. These films were cut to the size of the food block and placed on its upper surface. The films come in two 90 m m thick sheets (i.e. 0.18 mm in total), called ‘A’ and ‘C’, which release a red dye when pressed together. In the tests, the films were wrapped in a single layer of 10 m m thick plastic food wrapping to avoid damage by fluids (Liggins et al. 1995). For some foods, a coating of dark lipstick was painted on the inside of the finger-stall lying against the lower food surface or on the food surface itself (figure 1c). This served to mark the depth of incisal penetration prior to cracking. Each subject repeated the test once on each of the seven foods, which were given in random order. Afterwards, both the average maximum compressive stress against the incisors and the depth of incisal indentation were measured using an image analyser.
The films require user calibration because the extent of colour development, depending basically on the compressive stress, also varies to some degree with temperature, humidity and the rate and duration of loading (Liggins 1997). Temperature and relative humidity in the laboratory were controlled at 21 °C and 55–70%, respectively. The exact loading conditions during a bite probably varied between subjects and we did not measure the velocity of jaw movement in this experiment. Judging from the experiments of Kohyama et al. (2001), incisal velocities may reach 10 mm s2 1. However, we standardized conditions to a rapid loading rate held momentarily. We pressed a hemispherical indenter, attached to the crosshead of the universal testing machine, onto the films, moving it at 900 mm min2 1 to its target. We then stopped the crosshead to make a static indentation held for ca. 1 s duration at a variety of loads (measured by 10 or 50 N load cells). The indenter was then unloaded at the same crosshead speed and indentations measured with a Leica QWin (UK) image analyser. The surface area of the indentation was measured and divided into the load to obtain the average pressure. The optical density of dye release was obtained by reading the grey level obtained from image analysis. A log–log plot of grey level versus average pressure was linear within bounds close to those indicated by the manufacturer. The stress range for a film was, however, not much greater than an order of magnitude and two films with overlapping stress ranges, ‘low pressure’ and ‘medium pressure’, were required.
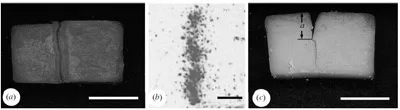
Figure 3. (a) Penetration of ‘extra sharp’ Cheddar produced by two central lower incisors, shown in top view. The part of the specimen to the right side was inserted in the mouth: the ‘shovel-shaped’ nature of the incisal edge, common in ethnic Chinese, is clearly seen. The lipstick stain shows the depth of indentation. Scale bar, 10 mm. (b) Colour development (shown as a grey area) of a ‘low pressure’ film produced during loading by one of these incisors. Scale bar, 1 mm. (c) A side view of the Cheddar specimen showing the depth of penetration, confirmed by inspection of lipstick staining, marked as a. A crack is seen extending from this indentation. Scale bar, 10 mm.
After each experiment, the finger-stall was opened and the films subjected to image analysis as described above. The average optical density was measured, from which the average compressive stress could be obtained by reference to the standard curve.
4. RESULTS
No surface slippage between food particle, film and finger-stall was observed during the tests. The extent of incisal penetration (figure 3a,b) was usually clear. The shape and extent of the red stain on the pressure-sensitive film was consistent with incisal morphology (figure 3a). Figure 4 shows the mean for all subjects of in vivo stressrelated measurements on the y-axis regressed against instrumental (in vitro) evaluations on the x-axis. A linear relationship, obtained by least-square regression, is clearly seen (r2 = 0.986; p < 0.001). The mean value of the slope, C4, was 14.64 (s.e. of 0.793). The y-intercept, 81.02 kPa m1 /2 (s.e. of 36.73), was not significantly different from zero ( p<0.08). Each point in figure 4 was subject to considerable inter-subject variation (shown by the s.d.), but each subject produced a linear response (table 1). For the mean of the two attempts by each subject, there was a significant relationship (r2 ranged between 0.70 and 0.97; p<0.02 or better) and the value of C4 varied approximately by a factor of three (table 1). The mean toughness and Young’s moduli of the foods, shown in table 2, were not correlated with each other (r = 20.02; p >0.9).
5. DISCUSSION
It appears that the theory of fracture applies just as well to a bite by the incisors at the entrance to the mouth as it does to the physical world away from the lips. The critical stress intensity factor, KIC, or its energetic equivalent (the square root of Young’s modulus times toughness), appears to be an appropriate characterization for these in relation to incision, fully supporting the psychophysical analysis of Vincent et al. (2002). Important features of foods are that their stress–strain curves are close to linearity. Nut kernels have a linear response and fail at small strains. In some of the other foods, the slope can decrease at higher strains, but it is very difficult to judge whether this is due to fracture (Charalambides et al. 1995). Carrot and nut particles fracture rather than yield. However, the fractured surfaces of foods, beyond the point of incisal indentation, did not show significant plastic distortion (figure 3c), implying that the assumptions made here are appropriate. Nevertheless, investigations on a broader scale could benefit from the nonlinear analysis developed by Purslow (1991) because this allows results from linear and nonlinear behaviours to be compared.
Vincent et al. (2002) suggest that mechanical characterizations of foods founded on sound theoretical support could replace the need for taste panels and psychophysical correlations in food texture studies, since mechanical tests are simpler and cheaper to run than taste panels and are free from cultural and linguistic limitations. However, it needs to be established that these tests do indeed match fracture conditions in the mouth. We argue that physiological investigations, such as those reported here, provide necessary in vivo confirmation. These experiments are so basic that food scientists could use the efficiency index to map the ease of biting on new products, even as part of a quality control regime. The experiments could be performed without a finger-stall, but it is difficult to preserve hygiene in experiments like this, even though the pressuresensitive films can apparently be sterilized (Liggins et al. 1994). The opacity of the finger-stall shielded subjects from recognizing the food visually and from any disturbing influence following flavour release after the bite, but a thinner finger-stall is perhaps indicated.
Dentists might use the method to establish the anatomical and physiological factors that influence the incisal efficiency index. The experiments here are relevant mainly to the lower incisors, but the procedure could be reversed with the film placed on the lower surface of the food particle. Then, with the particle held just above the lower incisors, the head could be moved to make a bite with the upper incisors. Thus, two efficiencies could be measured, one for the upper and the other for the lower incisors. However, establishing the parameters that influence these efficiencies requires a large sample of subjects drawn from a population, making impressions of the incisors and establishing their orientation. The combination of morphological factors that produce the lowest value of C4 , i.e. the lowest multiple of that which testing machine values generate, may define the optimum functional state. Such an investigation must surely be of significance in guiding the philosophy behind orthodontic treatments. The measurement of physiological variables must also play a part in such a survey. Prime among these must be the velocity of jaw movement and the strain rates that could be imposed on food particles. These are very difficult to investigate during mastication with the post-canine teeth, although the relatively invariant rhythm of jaw motion suggests that smaller particles suffer higher strain rates than larger ones. The strain rate may, of course, not have any general relationship to the speed of cracks that form as particles become fragmented (Lucas et al. 2002). However, all these factors are far more amenable to analysis during a first bite and could help to establish whether the quasi-static conditions assumed here are violated.
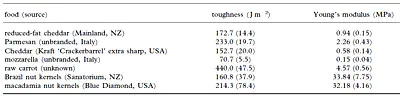
Table 2. Means and standard deviations of mechanical measurements on the test foods. (The sample size was 10 for each test.)
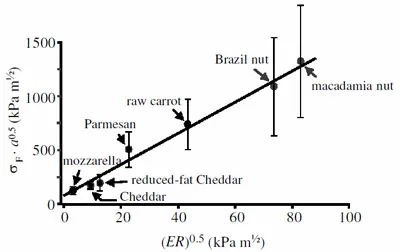
Figure 4. The mean for all subjects of the product of the average maximum in vivo compressive stress during incision and square root of penetration distance of the lower incisors is plotted on the y-axis against the square root of the product of Young’s modulus and toughness of food (both measured on a mechanical tester). A linear relationship, predicted in the text, is shown. Mean for all subjects r2 = 0.986; p<0.001.
The incisors of anthropoid primates vary greatly in their size, shape and occlusal relationships. There are several means by which mammals in general (Osborn et al. 1987), and primates in particular (Ungar 1992), ingest foods. Several of these involve the use of the teeth more as a way of gripping foods than as a direct means of fracture (figure 5). An example of this in primate feeding is ‘stripping’ (Osborn et al. 1987; Ungar 1994), which involves pulling leaves off a branch still attached to its parent plant. Primates also use their hands extensively when feeding, allowing relative movement between the hands and incisor teeth to produce some element of tension. The experiments reported here probably apply most closely to behaviour with fleshy fruits, particularly ‘peeling’ (Gautier-Hion et al. 1985; Leighton 1993) and ‘scraping’ categories. However, if all such food processing methods could be mimicked, then in combination with field measurement of the mechanical properties of these foods by primatologists (Lucas et al. 2001), studies on humans might have a critical part to play in explaining the evolution of incisor shape.
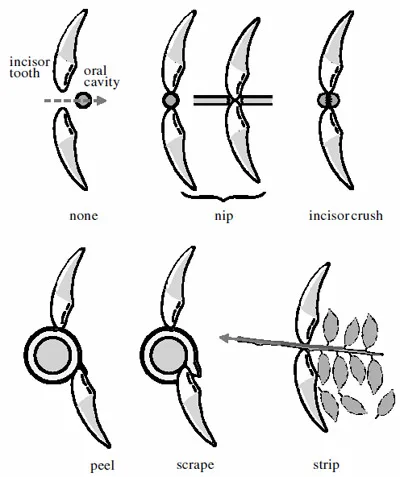
Figure 5. The various modes of ingestion employed by primates. Some involve the use of incisors to crack objects, while others involve them in grip. (Adapted from Osborn et al. (1987) and Ungar (1992) with permission.)
The authors thank the Committee for Research and Conference Grants (University of Hong Kong) for financial support.
REFERENCES
- Agrawal, K. R., Lucas, P. W., Prinz, J. F. & Bruce, I. C. 1997 Mechanical properties of foods responsible for resisting food breakdown in the human mouth. Arch. Oral Biol. 42, 1–9.
- Ashby, M. F. 1998 Checks and estimates for material properties. I. Ranges and simple correlations. Proc. R. Soc. Lond. A 454, 1301–1321. (DOI 10.1098/rspa.1998.0208.)
- Atkins, A. G. & Mai, Y.-W. 1985 Elastic and plastic fracture. Chichester: Ellis Horwood.
- Bourne, M. C. 2002 Food texture and viscosity, 2nd edn. New York: Academic Press.
- Charalambides, M. N., Williams, J. G. & Chakbrabarti, S. 1995 A study of the influence of ageing on the mechanical properties of Cheddar cheese. J. Mater. Sci. 30, 3959–3967.
- Darvell, B. W., Lee, P. K. D., Yuen, T. D. B. & Lucas, P.W. 1996 A portable fracture toughness tester for biological materials. Meas. Sci. Technol. 7, 954–962.
- Gautier-Hion, A. (and 11 others) 1985 Fruit characters as a basis of fruit choice and seed dispersal in a tropical forest vertebrate community. Oecologia 65, 324–337.
- Hylander, W. L. 1975 Incisor size and diet in anthropoids with special reference to Cercopithecidae. Science 189, 1095– 1098.
- Khan, A. A. & Vincent, J. F. V. 1993 Anisotropy in the fracture properties of apple flesh as investigated by crack-opening tests. J. Mater. Sci. 28, 45–51.
- Kohyama, K. & Nishi, M. 1997 Direct measurement of biting pressures for crackers using a multiple-point sheet sensor. J. Texture Stud. 28, 605–617.
- Kohyama, K., Nishi, M. & Suzuki, T. 1997 Measuring texture of crackers with a multiple-point sheet sensor. J. Food Sci. 62, 922–925.
- Kohyama, K., Sakai, T. & Azuma, T. 2001 Patterns observed in the first chew of foods with various textures. Food Sci. Technol. Res. 7, 290–296.
- Leighton, M. 1993 Modeling dietary selectivity by Bornean orangutans: evidence for integration of multiple criteria in fruit selection. Int. J. Primatol. 14, 257–313.
- Liggins, A. B. 1997 The practical application of Fuji Prescale pressure-sensitive film. In Optical measurement methods in biomechanics (ed. J. F. Orr & J. C. Sheldon), pp. 173–179. London: Chapman & Hall.
- Liggins, A. B., Hardie, W. R. & Finlay, J. B. 1994 Sterilization of Fuji pressure-sensitive film. Med. Eng. Phys. 16, 496–500.
- Liggins, A. B., Surry, K. & Finlay, J. B. 1995 Sealing Fuji Prescale pressure sensitive film for protection against fluid damage: the effect on its response. Strain 31, 57–62.
- Lucas, P. W., Beta, T., Darvell, B. W., Dominy, N. J., Essackjee, H. C., Lee, P. K. D., Osorio, D., Ramsden, L., Yamashita, N. & Yuen, T. D. B. 2001 Field kit to characterize physical, chemical and spatial aspects of potential foods of primates. Folia Primatol. 72, 11–15.
- Lucas, P.W., Prinz, J. F., Agrawal, K. R. & Bruce, I.M. 2002 Food physics and oral physiology. Food Qual. Pref. 13, 203–213.
- Osborn, J. W., Baragar, F. A. & Grey, P. 1987 The functional advantage of proclined incisors in man. In Teeth revisited: Proc. VII Int. Symp. Dental Morphology (ed. D. E. Russell, J. P. Santoro & D. Sigognean-Russell). Mem. Mus. Nat. Hist. Nat. Paris C 53, 445–458.
- Paphangkorakit, J. & Osborn, J. W. 1997 Effect of jaw opening on the direction and magnitude of human incisal bite forces. J. Dent. Res. 76, 561–567.
- Purslow, P. 1991 Notch-sensitivity of nonlinear materials. J. Mater. Sci. 26, 4468–4476.
- Ungar, P.S. 1992 Incisal microwear and feeding behavior of four sumatran anthropoids. PhD thesis, State University of New York at Stony Brook.
- Ungar, P. S. 1994 Patterns of ingestive behavior and anterior tooth use differences in sympatric anthropoid primates. Am. J. Phys. Anthropol. 95, 197–219.
- Vincent, J. F. V., Saunders, D. E. J. & Beyts, P. 2002 The use of stress intensity factor to quantify ‘hardness’ and ‘crunchiness’ objectively. J. Texture Stud. 33, 149–159.